Effect of Processing Conditions and Die Design on Die Drool Phenomenon for HDPE Polymer Melt¶
Martin Zatloukal1,2, Jan Musil1,2
1 Polymer Centre, Faculty of Technology, Tomas Bata University in Zlín, nám. T. G. Masaryka 275,
762 72 Zlín, Czech Republic
2 Centre of Polymer Systems, Tomas Bata University in Zlín, nám. T. G. Masaryka 5555,
760 01 Zlín, Czech RepublicAbstract
In this work, the die drool phenomenon analysis has
been performed for HDPE polymer melt by using specific
type of experimental set-up. It has been revealed that the
thermally induced degradation occurring inside the
processing equipment may leads to HDPE melt
viscosity/elasticity enhancement, which promotes
unwanted material accumulation at the end of the
extrusion die. It has been found that for particular
processing conditions, shaped die, flared die or the use of
hard chrome instead of stainless steel can reduce die drool
phenomenon for HDPE.
Introduction
Die drool, also called die build-up, die bleed or plateout,
is undesirable spontaneous accumulation of polymer
melt at the exit edges or open faces of extrusion die during
melt extrusion of polyolefins, PVC, or filled polymers.
This unwanted phenomenon can occur in all common
extrusion processes (film blowing, pipe or profile
extrusion, fiber spinning, film casting etc.) and after it
arises, the productivity of extrusion line rapidly decreases
from following basic reasons. Firstly, small amount of
accumulated and usually degradated material sticks to the
extrudate at the die exit region which cause depreciation
of the final product. In the case of film casting or
extrusion of solid profiles is possible to solve this problem
only by strip the accumulated material from the die exit
region. However, in the case of film blowing, pipe, cored
profile extrusion or wire sheathing the die drool arises not
only at the outer exit region but also at the inner one
thereby the first clean opportunity is impossible.
Therefore, in these cases the extrusion line is necessary to
periodically switch off and after cleaning the die which is
uneconomical and time consuming.
The fundamental problem of die drool phenomenon is
to understand and describe the formation principle of this
phenomenon. In the open literature, several factors have
been found to support the formation of this instability.
They include pressure fluctuations in screw [1], volatitilies, low molecular fractions of the polymer, fillers,
poor dispersion of pigments [2], die swell [1, 3],
processing near degradation temperature [4], dissimilar
viscosities in blends [5], die design [6, 7, 8] etc.
At the present time, two basic types of die drool
phenomenon are known: external and internal. It has been
recently found that the external type of die drool
phenomenon for metallocene type of LLDPE polymer is
predominantly driven by the extrudate free surface rupture
and suction effect due to negative pressure generated at
the die exit region [9, 10] which can be suppressed by the
die exit angle modification [9, 10]. On the other hand, the
internal type of die drool phenomenon can be caused by
the flow induced fractionation occurred before the
polymer melt leave the extrusion die which is not fully
understood yet [11]. Thus, the effect of processing
conditions and die design on this unwanted flow
phenomenon has been investigated in this work for unfiled
HDPE extrusion through annular extrusion die.
Experimental
Material characterization
In this work, HDPE Tipelin FS 450-26 (TVK,
Hungary) polymer, widely used in the film extrusion was
chosen for the experimental research. The basic material
characteristics are provided in Table 1 and [12]. In order
to evaluate the HDPE polymer thermal stability, firstly,
polymer pellets were added and melted in the Rosand
RH7-2 control speed capillary rheometer equipped by
capillary die having 16mm length and 1mm radius. After
each 10 minutes of the rest, the constant shear rate (80 s
-1)
has been applied and the equilibrium capillary pressure
drop has been measured. This procedure was repeated 30
times to cover 5 hour degradation time period after which
the extruded polymer has been collected. This procedure
was applied for two different melt temperatures T = 210
oC and T = 260
oC. Effect of processing temperature and time
on the extrusion pressure for the tested HDPE sample is
provided in Figure 1. It is clearly visible that the extrusion
pressure rise occurs during the time suggesting that
temperature induced degradation leads to molecular weight increase of the tested polymer and the effect is
much more pronounced for the higher temperature.
Die drool measurements
The die drool experiments were carried out on a
conventional Brabender Plasti – Corder 2000 single-screw
extruder with diameter D = 30 mm and L = 25 D (standard
single-thread screw with compression ratio 4:1, and
lengths of zones: feed L
1 = 10D, compression L
2 = 3D, metering L
3 = 12 D) which was included in the laboratory extrusion line. The schematic view of laboratory extrusion
line is depicted in Figure 2. This line consists of extruder
with four heating zones, transition annular part, specially
designed extrusion die, which is introduced in [9, 10],
photo camera placed near the die exit, and draw-off
mechanism. In our experiments, two different processing
conditions were used. In the first case, the extruder zones
(from the hopper to the die) were heated to
T
1 = 80°C, T
2 = 200°C, T
3 = 240°C and
T
4 = 260°C whereas in the
second case, third and fourth extruder zone temperatures
were decreased to T
3 = T
4 = 210°C by keeping the annular
tube (connecting die and extruder) and die exit
temperature in both cases constant, T
5 = T
6 = 150°C. Die
drool accumulation has been investigated for both
temperature profiles along the screw and constant mass
flow rate (1.1 kg.hr
-1). In this study, following extrusion
dies were utilized: straight die, shaped die, flared die
manufactured from stainless steel and straight die
manufactured from hard chrome material (see Figure 5 for
more detail). For each individual test, barrel, screw and all
parts of the die have been perfectly cleaned to ensure the
reproducibility of the performed measurements.
Results and discussion¶
The character of the die drool phenomenon for HDPE
within the time has been investigated for the following
processing conditions at which the die drool intensity was
found to be the highest: temperature profile along the
screw: T
1 = 80°C, T
2 = 200°C, T
3 = 240°C, T
4 = 260°C;
annular tube (connecting die and extruder) and die exit
temperature T
5 = T
6 = 150°C; mass flow rate = 1.1 kg.hr
-1.
The obtained results are depicted in Figure 3. It is clearly
visible that the accumulated material takes a torus shape
along the exrudate surface rather than flakes or powder as
in the case of external mLLDPE die drool phenomenon
[9-10]. Moreover, no surface defects occur during the
HDPE die drool accumulation which lead us to the
conclusion that rupture of the extrudate free surface at the
die exit is not driving factor in this case (unlike for
mLLDPE [9-10]).
The effect of temperature profile along the screw
channel on the die drool intensity is visualized in Figure 4
for constant mass flow rate. It is nicely visible that the last
two screw zone temperature increase from 210°C to 260°C causes significant die drool intensity increase even if the
annular tube (connecting die and extruder) and die exit
temperatures remains to be unchanged, equal to 150°C.
This behavior can be explained by the increased
HDPE melt viscosity/elasticity due to thermally induced
degradation (as showed in the performed rheological
analysis) promoting flow induced fractionation of low
molecular weight component of HDPE, which is in good
correspondence with recent work on molecular weight
fractionation during polymer processing [11] and
theoretical study of internal die drool phenomenon [10]. In
more detail, the studied die drool phenomenon can be
understood through originally Busse’s hypothesis [13]
stating that during the flow, larger (highly elastic) chains
acquire more elastic energy near the wall than small
molecules which generates thermodynamic force tending
to increase the concentration of small molecules at the
wall (area of the high stress), and of the larger chains at
the centerline (area of the low stress). In the light of this
hypothesis, (where die drooled material can be viewed as
the low molecular weight component of HDPE), increased
die drool phenomenon through melt elasticity
enhancement can be explained by the increased
thermodynamic force driving molecular weight
fractionation intensity during the flow.
The effect of die design and die material on the die
drool phenomenon for HDDPE polymer melt has also
been investigated in this work. It was found that for the
particular processing conditions and given time period, the
stainless steel shaped die (22.2% die drool reduction),
stainless steel flared die (46% die drool reduction) or the
use of hard chrome straight die (20.2% die drool
reduction) reduces die drool phenomenon with respect to
reference stainless steel straight die. Die drool shape for
the reference stainless steel straight die and the most
stabilizing flared die is visualized in Figure 6.
Conclusion
In this work, the die drool phenomenon analysis has
been performed for HDPE polymer melt by using specific
type of experimental set-up. It has been revealed that the
temperature increase at the end of the extruder, by keeping
annular tube (connecting die and extruder) and die exit
temperature unchanged, leads to die drool intensity
increase. It has been proved that this type of the internal
die drool phenomenon is caused by the thermally induced
HDPE degradation enhancing melt elasticity and thus
promoting the flow induced fractionation of the HDPE
melt. It has also been found that for particular processing
conditions, shaped die, flared die or the use of hard
chrome instead of stainless steel can reduce die drool
phenomenon for HDPE.
Acknowledgement
The support of the projects by the Ministry of
Education CR (KONTAKT ME08090, MSM
7088352101) are gratefully acknowledged.
References
1. I. Klein, Plastics World, 112 – 113, (May 1981).
2. S. J. Kurtz, and S. R. Szaniszlo, U.S. Patent 5,008,056
(1991).
3. T. A. Hogan, P. Walia and B. C. Dems, Polym. Eng.
Sci. 49, 333-343 (2009).
4. D. A. Holtzen and J. A. Musiano, SPE RETEC, St.
Louis, (1996).
5. D. E. Priester, SPE ANTEC Tech. Papers, 40, 2059
(1994).
6. J. D. Gander and J. Giacomin, Polym. Eng. Sci. 37,
1113-1126 (1997).
7. F. Ding, L. Zhao, A.J. Giacomin and J. D. Gander,
Polym. Eng. Sci. 40, 2113-2123 (2000).
8. P. K. Dhori, R. S. Jeyaseelan, A. J. Giacomin and J. C.
Slattery, J. Non-Newtonian Fluid Mech.71, 231-243
(1997).
9. K. Chaloupkova and M. Zatloukal, Polym. Eng. Sci. 47,
871-881 (2007).
10. K. Chaloupkova and M. Zatloukal, J. Appl. Polym.
Sci. 111, 1728-1737 (2009).
11. M.D. Shelby, G.B. Caflisch, Polym. Eng. Sci. 44(7),
1283-1294 (2004).
12. R. Pivokonsky, M. Zatloukal, P. Filip, J. NonNewtonian
Fluid Mech., 150(1), 56-64 (2008).
13. W.F. Busse, Physics Today, 50, 32-41 (September
1964).
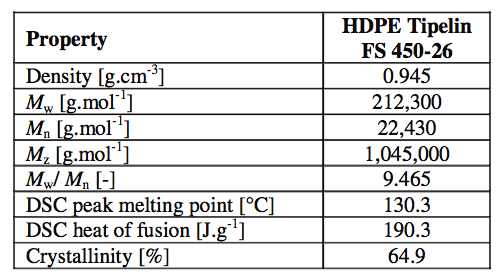
Table 1: Basic characterization of chosen polymer.
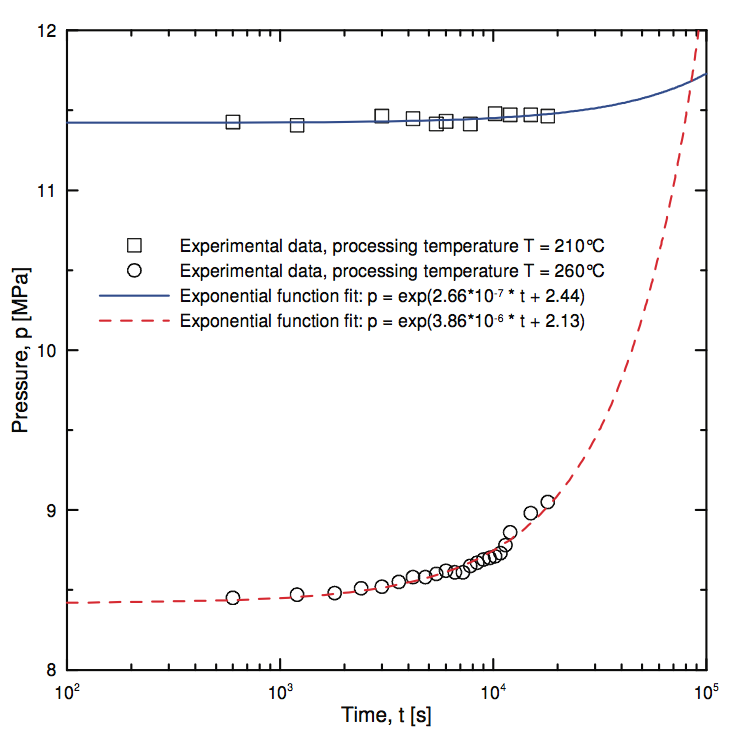
Figure 1. Effect of processing temperature on the time dependent extrusion pressure for tested HDPE Tipelin FS 450-26 sample.
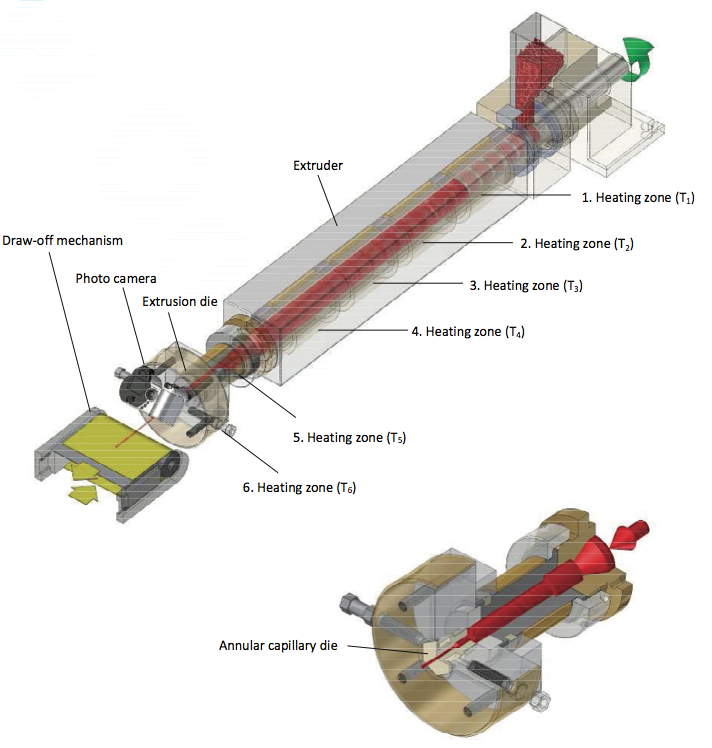
Figure 2. 3D sketch of laboratory extrusion line (top) together with detail view of the extrusion die (bottom).
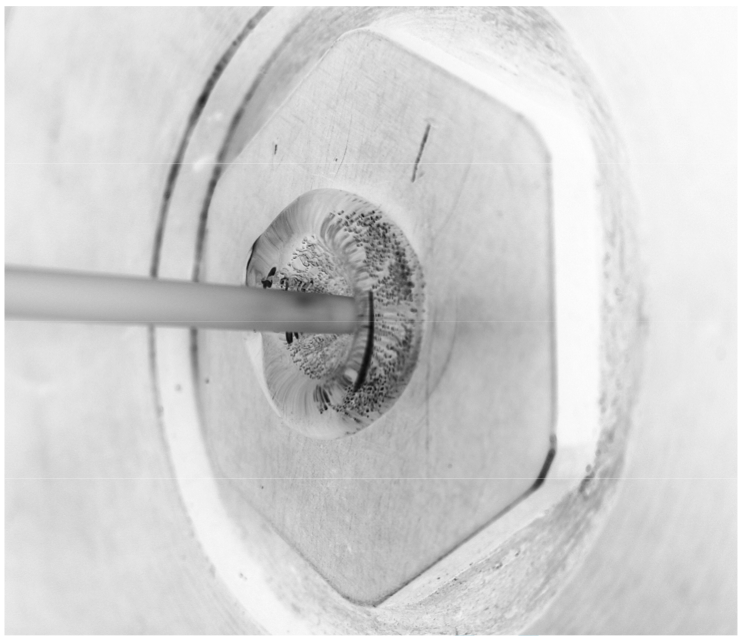
Figure 3. Die drool visualization at the die exit for the tested HDPE sample after 15 min of extrusion (temperature profile along the screw: T
1 = 80
oC, T
2 = 200
oC, T
3 = 240
oC, T
4 = 260
oC; annular tube (connecting die and extruder) and die exit temperature T
5 = T
6 = 150
oC; mass flow rate = 1.1 kg.hr
-1).
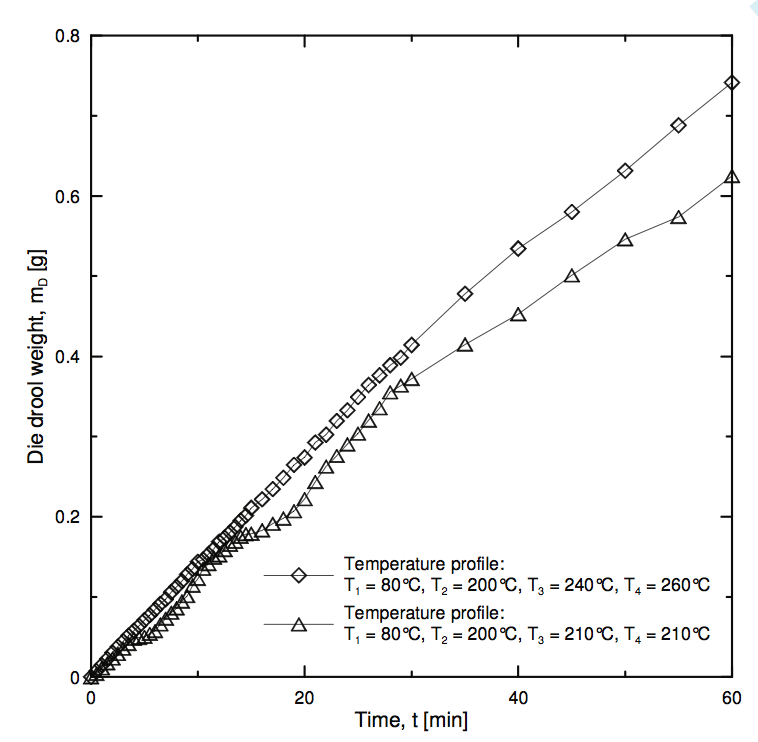
Figure 4. Die drool amount as a function of time for two different temperature profiles along the screw; mass flow rate = 1.1 kg.hr
-1, annular tube (connecting die and extruder) and die exit temperature T
5 = T
6 = 150
oC.
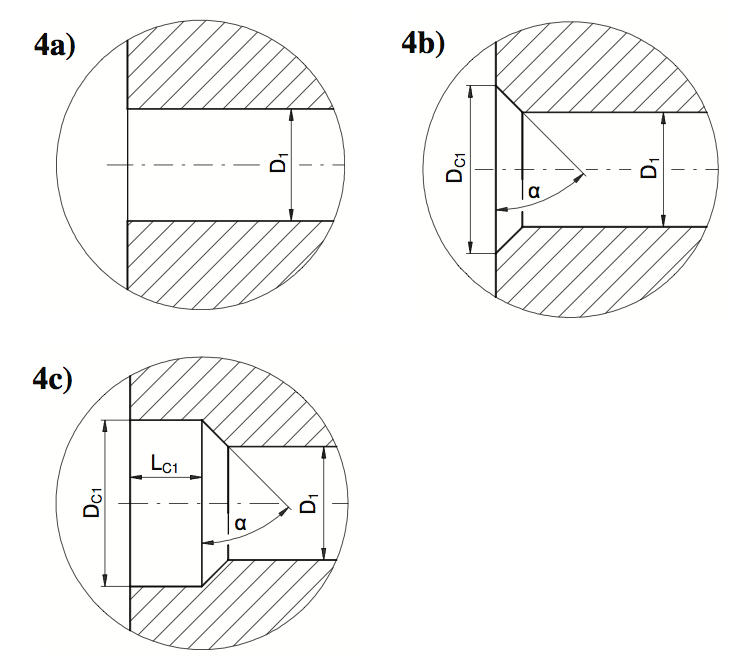
Figure 4. Investigated capillary dies, D
1=1.6mm, alpha=45°. 4a) Straight die; 4b) Shaped die, D
C1=2mm; 4c) Flared die, D
C1=2mm , L
C1=12mm.
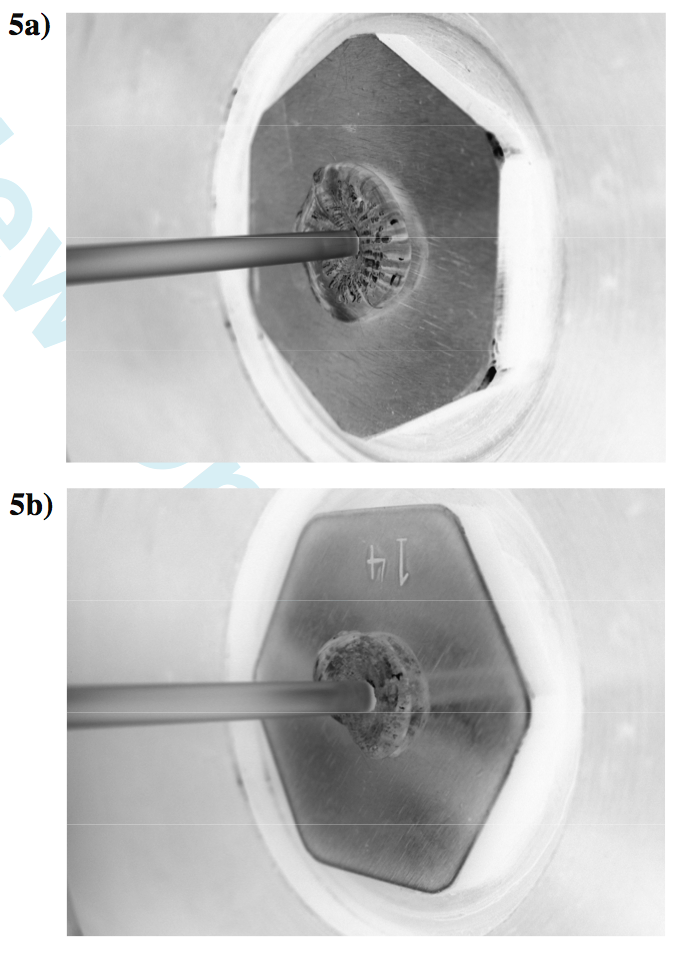
Figure 5. Visualization of die drool phenomenon at the die exit after 10 minutes of extrusion. 5a) Stainless steel straight die; 5b) Stainless steel flared die (temperature profile along the screw: T
1 = 160
oC, T
2 =165
oC, T
3 =170
oC, T
4 = 170
oC; annular tube (connecting die and extruder) and die exit temperature T
5 = T
6 = 170
oC; mass flow rate = 0.9 kg.hr
-1).
Return to
Paper of the Month.