Troubleshooting and Mitigating Gels in Polyolefin Film Products¶
Mark A. Spalding, The Dow Chemical Company, Midland, MI
Eddy Garcia-Meitin and Stephen L. Kodjie, The Dow Chemical Company, Freeport, TX
Gregory A. Campbell, Clarkson University/Castle Associates, Jonesport, ME Abstract
The term “gel” is commonly used to refer to any small
defect that distorts a film product. Eliminating gel defects
from extruded polyolefin film products can be difficult,
time consuming, and expensive due to the complexity of
the problem and the high levels of off-specification product
produced. This paper discusses the identification of gel
types, the common root causes for gels, and the technical
solutions for mitigating gels in film products produced
using single-screw extruders.
Introduction
Troubleshooting extrusion processes where gels are
appearing in polyethylene (PE) film products can be
difficult due to the number of different gel types that are
possible. For these processes, the troubleshooter must be
able to diagnose the problem quickly and provide an
economically viable technical solution [1]. Because gels
can originate from numerous sources, the troubleshooter
must be able to identify the characteristics of the gel and
recognize the likely possibilities of the source. Process
changes must then be performed to mitigate the gel defects.
There are many types of gels [2] and the most
common include: 1) highly oxidized polymeric material
that appears as brittle black specks, 2) polymeric materials
that are crosslinked via an oxidative process, 3) highlyentangled
polymeric material (such as high molecular
weight species) that are undispersed but not crosslinked, 4)
unmelted resin, 5) filler agglomerates from masterbatches,
and 6) a different type of resin or contaminant such as
metal, wood, cloth fibers, or dirt. A crosslinked resin gel is
typically formed during an oxidation process, resulting in
the crosslinking of the resin chains and the generation of
discolored gels. Highly-entangled gels are typically high
molecular weight polymer chains that are entangled and
thus difficult to disperse during the extrusion process.
When analyzed using a hot stage microscope, this gel type
will melt as the stage temperature is increased. When the
stage temperature is then decreased, the gel will crystallize,
creating the appearance of a gel as a solid polymer
fragment. Since these gels are not oxidized they are not
associated with color. They are commonly referred to as
undispersed or unmixed gels. Unmelted resin exiting with
the discharge can sometimes occur, especially at high
extrusion rates. These gels will melt during heating with a
hot-stage microscope, and typically they will not reform
during the cooling phase. Numerous sophisticated methods
are available for analyzing gels including epi-fluorescence
microscopy, polarized light microscopy, and electron
microscopy with x-ray analysis. These methods are
discussed in the next sections.
Gels can be generated from many different sources
and include: 1) the resin manufacturer, 2) the converting
process, 3) pellet blending of resins with significantly
different shear viscosities, 4) pellet blending of different
resin types, and 5) direct contamination. Modern resin
manufacturing processes exclude oxygen from the system
and are very streamline such that process areas with long
residence times do not exist. As such, crosslinked and
oxidative gels are likely not generated by the manufacturer.
Improperly designed extrusion equipment and processes,
however, are common, leading to the oxidative degradation
of resins and crosslinked gels. Several case studies in the
next sections show how poorly designed processing
equipment can lead to crosslinked and unmixed gel
contamination of film products.
The goal of this paper is to describe the different type
of gels that are likely to occur in polyolefin film products,
techniques for identifying the gel type, and technical
solutions to mitigate them from single-screw extrusion
processes.
Protocols for Gel Analysis
Established protocols for gel analysis in polymer films
are well documented in the literature [2-4]. For example,
gels can be identified using the schematic process [4]
shown in Figure 1. Typically a film with defects is visually
inspected using a low power dissecting microscope. The
gels can be classified based on size, color, and shape, and
isolated using a razor blade or scissors. Cross sections of
the gels ranging from 5 µm to 10 µm thick are collected at
temperatures below the glass transition temperature (T
g) of
the film using a cryogenic microtome; i.e., -80°C to -
120°C. For optical examination, a thin section containing
the gels is placed on a glass microscope slide with a drop
of silicon oil and covered with a glass cover slip.
Additional sections are collected for examination via hot
stage microscopy and for compositional identification if
needed.
After collecting the sections, the polished block-face
containing the remainder of the gel is retained. In many
instances, gels arise from inorganic contaminants such as
the metallurgy from pellet handling equipment, extruders,
or components from masterbatches. Examination of these
inorganic components are best performed with the blockface
sample using a scanning electron microscope (SEM)
equipped with an energy dispersive x-ray detector (EDX)
[5,6]. In some cases, additives or inorganic residues are
present in low concentrations within the gels. A method to
enrich the concentration of these materials is to expose the
block-face containing the gel to oxygen plasma. Etching
will preferentially remove the polymer at a much faster
rate than the inorganic materials, enriching the inorganic
components for elemental analyses. It must be noted that
prior to SEM and EDX analyses, a thin conductive coating
like carbon is typically evaporated onto the sample to
render it conductive under the electron beam.
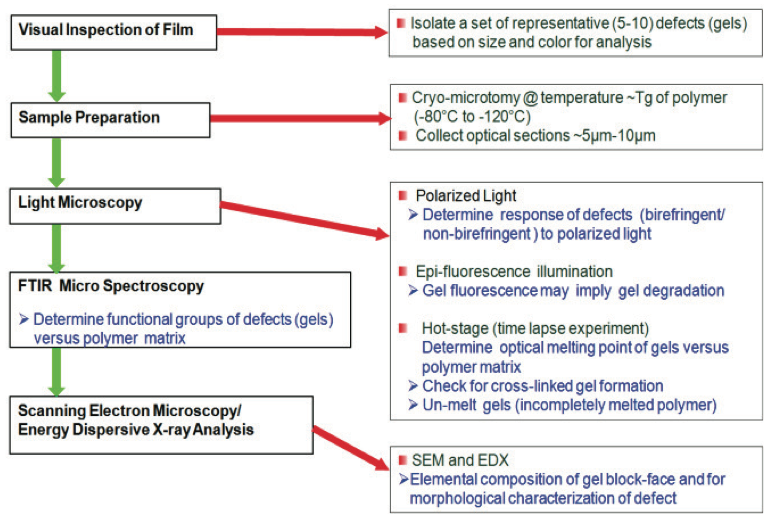
Figure 1. Methodology for characterizing defects in polymer films [4].
The next sections will demonstrate various methods of
analysis used for common gel types.
Oxidized Gels
The most common type of gel is caused by oxidative
processes that crosslink the PE chains. The best way to
identify this gel type is by observing them with polarized
light and ultraviolet (UV) light sources. Transmitted
polarized light microscopy represents an effective
technique [7] that can be used to investigate structures in
crystalline films. For example, black speck gels were
contaminating a multilayer film product. The gels were
relatively brittle when cut for analysis. The source was
unknown. The detail of a gel is clearly visible using
transmitted polarized light, as shown in Figure 2a. Close
examination of this gel using epi-fluorescence with an ultraviolet light source caused an intense fluorescence
emission, as shown in Figure 2b. This type of emission
suggests thermal oxidation and crosslinking of the
polymer. Micro-infrared analysis of the gel indicated that it
contained oxidized PE and maleic anhydride, as shown by
the spectrum in Figure 3 (for clarity, this figure can be
found at the end of this paper). This material likely formed
on the metal surfaces of the extruder and then flaked off
during a minor process instability. The material then
flowed downstream and contaminated the film as a gel.
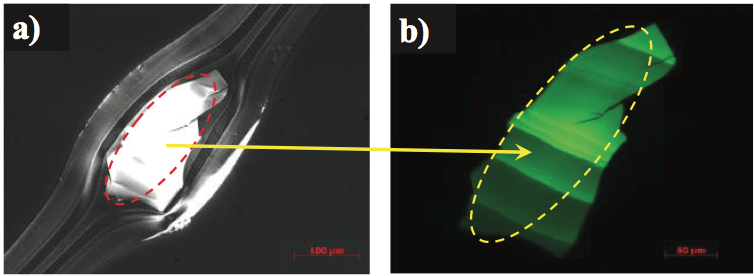
Figure 2. Transmitted polarized light images of a thermally oxidized and crosslinked gel in a multilayer film: a) photograph in polarized light, and b) the gel fluorescing under UV light.
Crosslinked Gels
Crosslinked gels are oxidized gels, but the level of
oxidation may not be enough to cause them to fluoresce
under UV light. These gels may have a level of
crystallinity and thus be birefringent under polarized light.
For example, the slightly birefringent gel shown in Figure
4a was studied using a temperature programmable hot
stage, polarizing light microscope [7]. The optical melting
temperature (T
m) of the gel was measured at 128°C and
consistent with the PE used to make the product, as shown
in Figure 4b. To determine if the gel was unmixed (highly
entangled but not crosslinked), the gel was held above the
melting temperature (135°C) and then stressed. A dental
tool was used to stress the top of the glass cover slip.
Crosslinked gels will appear birefringent, (Figure 4c) in
response to the anisotropy of stress distribution in the gel
to polarized light. The gel dimensions and shape remained
after cooling verifying crosslinking, as shown in Figure 4d.
If the gel was highly entangled and not crosslinked, the gel
would have disappeared after the stress and cooling were
applied.
Gels from Foreign Contamination
The origin of defects causing discoloration in
polyolefin pellets can be identified using light and electron
microscopy. For example, PE pellets from an in-plant
recycle re-pelletizing process contained pellets that were
off color and had black specks, as shown in Figure 5a. One
of these defects was isolated using the cross sectioning technique, as shown in Figure 5b. The cross section
revealed an intense reddish particle that caused the
discoloration of the pellet.
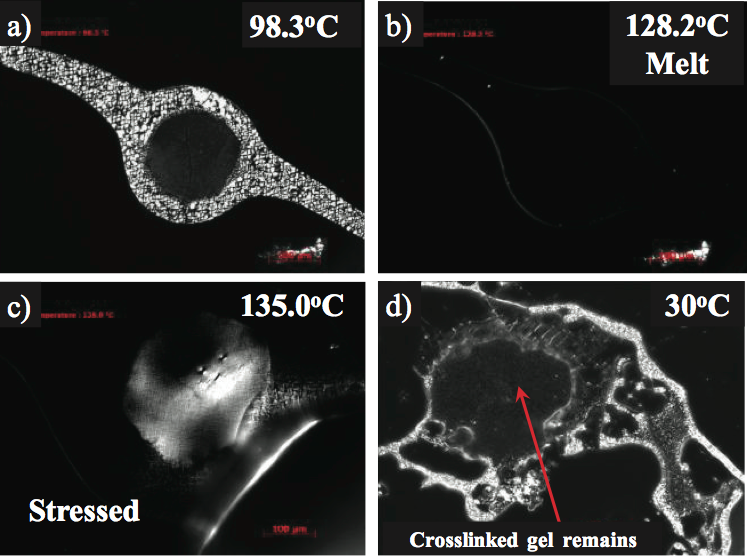
Figure 4. Hot stage microscopy of a crosslinked gel in a crystalline monolayer film: a) below the melting temperature, b) optical melting point at 128°C, c) appearance of birefringence after stressing at 135°C, and d) intact crosslinked gel after cooling to 30°C.
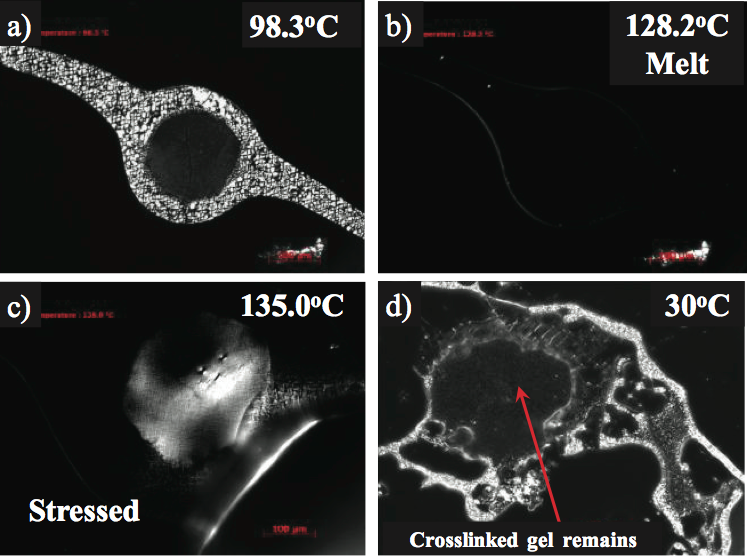
Figure 5. Photographs of foreign contamination in pellets of a re-pelletized reclaim stream: a) photomicrograph of discolored polyolefin pellets containing dark defects, and b) transmitted polarized light micrograph of a pellet cross section containing a defect.
SEM and EDX microanalysis were used to determine
that the defects contained primarily iron and oxygen, and it
likely was iron oxide. Figure 6 shows a backscatter
electron image (BEI) of the pellet block-face sample
showing the defect causing the discoloration and the
elemental spectrum. Metallic based defects can originate
from process equipment, railcars used for shipment, pellet
transfer lines, and poor housekeeping. The origin of the
iron oxide was likely from a storage bin.
In another example, a multilayer film product was
experiencing occasional gels. The gels were isolated and
the cross sections were collected as shown in Figure 7a.
These gels contained highly birefringent particles that resided in the core layer. The outer film layers appeared
amorphous and the core layer was slightly birefringent.
The optical melting temperature of the core layer was
determined to be 123°C while the birefringent gels melted
at 265°C. The melting temperature of 123°C was consistent
with the PE resin used to produce the core layer. The
higher melting temperature material and micro-infrared
analyses of the defects indicate that they were foreign
contaminants, and they were identified as a polyester resin.
The polyester resin was used in another process in the
converting plant, and it inadvertently contaminated the PE
feedstock.
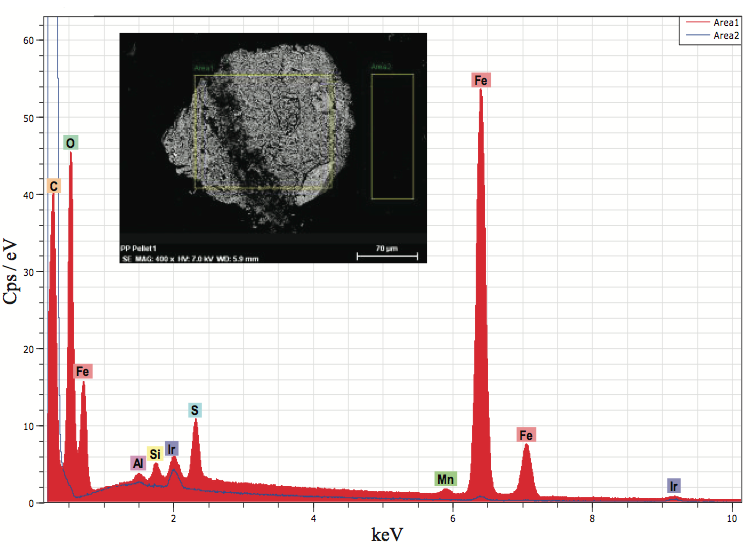
Figure 6. EDX microanalysis of an inclusion in a polyolefin pellet cross section (Figure 5b). The analysis indicated that the particle was likely iron oxide.
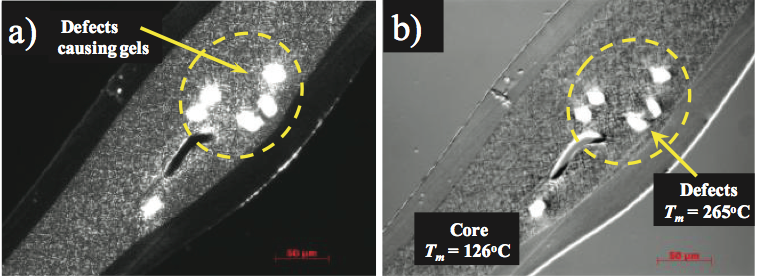
Figure 7. Photographs of gels in the core layer of a three layer film: a) transmitted polarized light, and b) hot stage microscopy was used to determine the melting temperatures of the core resin and defects.
Another common contaminant that produces gels is
fibers, as shown in Figure 8. In many cases, these
contaminants are cotton fibers from clothing and gloves or
cellulosic fibers from packaging materials. Fourier
transform infrared (FTIR) spectroscopy is one of the best
techniques for determining the chemical functionality of
organic based defects in PE films. The infrared absorbance
characteristics of the defect were determined using FTIR spectroscopy, as shown in Figure 9 (for clarity, this figure
can be found at the end of this paper). The broad
absorption bands near 3600 cm
-1 to 3100 cm
-1 are due to
hydroxyl (-OH) stretching vibrations, the C-H vibration
stretch is near 2916 cm
-1 to 2851 cm
-1, and the ester
carbonyl group absorption is near 1734 cm
-1. Based on the
infrared absorption characteristics, the defect in the PE
film is a cellulosic fiber with degraded PE resin.
Once the contaminant is identified, the troubleshooter
must determine how the material entered the feedstock
stream. Process controls must be identified and
implemented to mitigate the contaminant source.
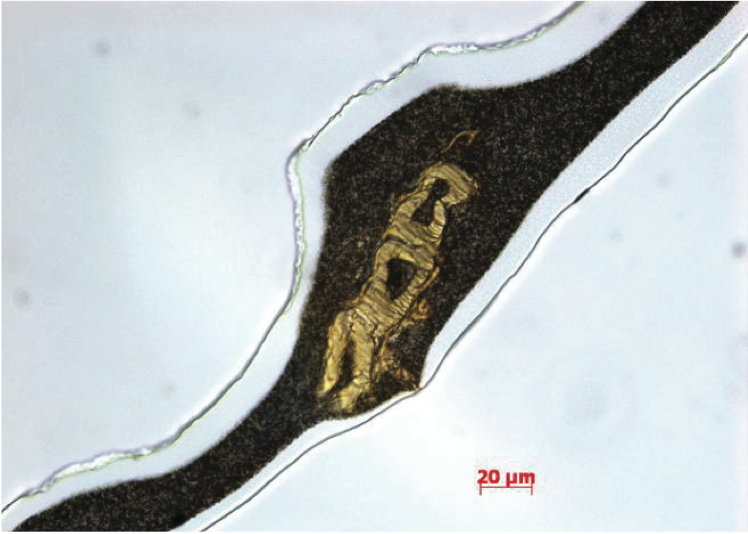
Figure 8. Transmitted bright-field image of PE film containing a fibrous gel.
Case Studies
Oxidized gels, carbon specks, and unmixed gels can
be created inside the extrusion processing line. Crosslinked
gels and black specks occur due to regions in the process
that are stagnant and have very long residence times in the
extruder. Unmixed gels and solid polymer fragments occur
because the resin was not subjected to a high stress level
during processing. This section provides several case
studies where these types of gels occurred. The technical
solutions to mitigate the gels are then presented.
Gel Showers in a Cast Film Process
Crosslinked gels can form in stagnant regions of screw
channels, transfer lines, and dies. The time required for
these gels to form range from about 30 minutes for linear
low density polyethylene (LLDPE) resin up to 12 days for
low density polyethylene (LDPE) resin. Stagnant regions
can occur at entries and exits of mixers [1] and barrier
sections, and they can occur when the metering channel of
smooth-bore extruders is not controlling the rate. In these cases, a section upstream of the metering section is rate
limiting, causing portions of the metering section to
operate partially filled [8,9]. When these channels operate
partially filled the main flow is on the pushing side of the
channel while the trailing side operates void at first. After a
period of time, clean resin gets into the void regions and
rotates with the screw for long durations. Eventually the
resin will degrade, forming crosslinked gels. Slight process
upsets can dislodge this material, allowing the material to
flow downstream creating a gel shower in the film.
A film plant was extruding a LDPE resin into a
specialty product using a cast film process [8,9]. Due to
high demand, a new 88.9 mm diameter, 33 L/D extruder
was installed in the plant. Soon after startup the product
was acceptable and high quality. After 12 days, the line
began to experience intermittent discharges of crosslinked
material (gel showers) and carbon specks. Photographs of
these gels are shown in Figure 10. In some cases, the gel
showers were observed 2 to 3 times per day and would last
from 1 to 5 minutes. The gels were clearly crosslinked and
were brown in color. The extrudate temperature was higher
than expected for the process. The intermittent gels
resulted in production downtime due to purging and in
numerous customer complaints. A high and costly level of
quality control was required to remove the gel
contaminated product from the prime product. Due to the
high amount of downtime and the high levels of quality
control needed, the operation of the new line was
considerably more expensive than planned.
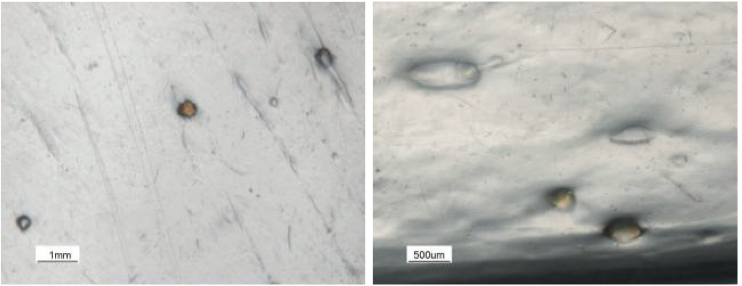
Figure 10. Photographs of crosslinked gels in a LDPE film.
It was hypothesized that the extruder was operating
partially full due to the low specific rate during operation.
To determine if partially filled channels were the root
cause of the reduced rate, high discharge temperature, and
degraded material, screw rotation was stopped and the
screw was removed while hot from the extruder.
Examination of the polymer on the screw indicated that in
the meter section about half of the channel width on the
trailing sides of the flights for all but the last diameter were
filled with a dark colored, partially carbonized LDPE resin,
indicating that these regions were stagnant. The reduced
flow rate caused these regions to be partially filled,
creating void regions on the trailing side of the channel.
Some of the resin adhered to the trailing side of the screw in the void regions and stayed there for extended time
periods, as shown in Figure 11. The resin adhering in the
void regions eventually degraded into the dark-colored,
crosslinked material. Small process variations dislodged
some of this material and caused the intermittent gel
showers that contaminated the product. Moreover,
compacted solids were found wedged in the channel at the
entrance to the barrier section. The wedged material was
caused by the relatively large width of the entering solid
bed being forced into the continually decreasing width of
the solids channel of the barrier section.
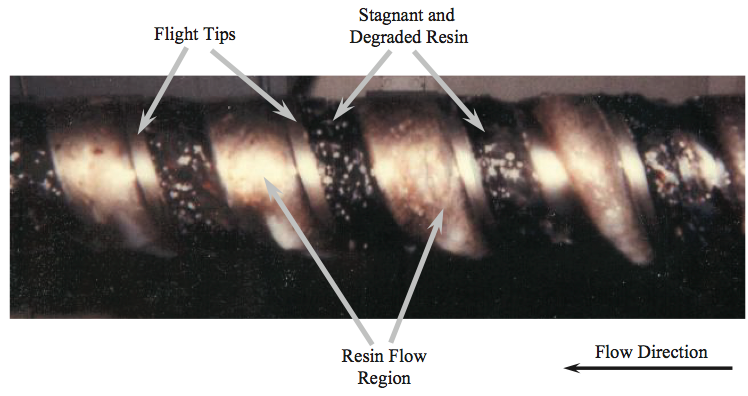
Figure 11. Photograph of a removed screw showing the resin flow and degraded resin due to stagnant regions [9].
The technical solution to eliminate this problem was a
simple modification to the entry of the barrier melting
section. For this modification [8], some of the metal in the
melt conveying channel was removed along with a portion
of the barrier flight, allowing some solid material to enter
the melt channel and reducing the restriction at the entry.
By reducing the restriction, the rate limiting step of the
process changed from the entry region of the barrier
section to the metering section. After the modification was
made, the gels were eliminated from the process.
Unmixed Gels
As stated previously, unmixed gels are highly
entangled species that are molten when they are discharged
from the die, but solidify first upon cooling to produce a
gel that appears as a solid polymer fragment. These types
of gels are easily removed from an extrusion process by
subjecting all molten resin to a one-time high level of
stress near the discharge of the extrusion screw. This stress
is easily applied using a Maddock-style mixer with a
relatively tight clearance on the mixing flight.
A film process was producing a monolayer film that
had a low level of gels. The gels were tested using hot
stage microscopy and identified as highly entangled
species (unmixed gels). These gels melted and then disappeared when heated and stressed via pressure
smearing using a dental tool, as shown in Figure 12.
The unmixed gels were removed by increasing the
stress level in the Maddock mixer. The stress level was
increased by decreasing the clearance on the mixing flight.
The stress level required to disperse unmixed gels depends
on the resin and the level of chain entanglement. In past
experiences, the stress level required to disperse PE
unmixed gels is about 100 to 200 kPa.
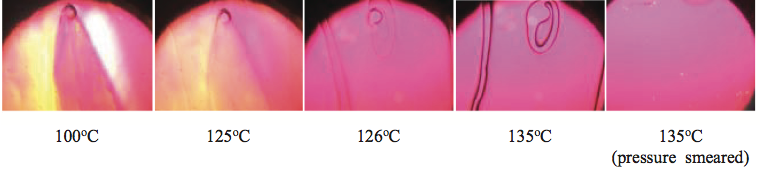
Figure 12. Photographs of an un-mixed gel at select temperatures using a hot-stage microscope. The un-mixed gel melted at about 135
oC. When the gel was smeared by moving the glass cover slip, the stress was enough to disentangle the polymer chains such that the gel would not reappear upon cooling.
A similar problem with solid polymer fragments
occurred for a thermoplastic polyurethane (TPU) resin
[10]. For this case, a combination of a lower compression
ratio, a longer barrier section with a very small barrier
flight clearance, a Maddock mixer with a small mixing
flight clearance, and deeper metering channels allowed the
TPU resins to extrude at twice the rate and provide high
quality extrudates that were free of solid polymer
fragments.
The shear stress that the material experiences for flow
across the mixing flight of the Maddock mixer can be
estimated using Equations 1 and 2. The shear stress level is
responsible for breaking up the entangled species. This
calculation is based on screw rotation physics [1].
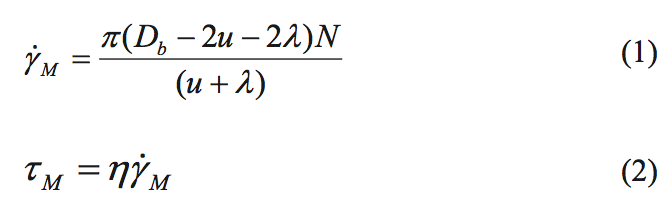
where Y
M is the average shear rate for flow over the
mixing flight in 1/s, N is the screw rotation rate in
revolutions/s, ή is the shear viscosity at the temperature of
the mixing process and at shear rate Y
M, D
b is the barrel diameter, u is the undercut distance on the mixing flight, λ
is the main flight clearance, and t
M is the shear stress that the material will experience for flow over the mixing
flight.
Carbon Specks in a Film Product
Carbon specks can be generated in the extruder
channels and in downstream transfer lines and dies if
stagnant regions are present. In general, these regions are
not very large like those in Figure 11. Instead, they are thin
coverings that occur at the flight radii or at entry and exits
of mixing devices [1]. In general, the region will first
create small crosslinked type materials that adhere to metal
surfaces. With additional residence time, the crosslinked
material will form a thin carbon layer of highly oxidized
material. When the layer breaks away from the metal, it is
discharged as black specks in the PE film. These specks
will fluoresce under UV light.
A LLDPE blown film line was experiencing black
specks in the product. In order to locate the source, a
Maddock solidification experiment [11] was performed
where a small amount of a red color concentrate was added
to the feedstock resin, after the red color appeared in the
extrudate screw rotation was stopped, and the resin was
solidified in the channels. A photograph of the
experimental sample [12] is shown in Figure 13. Here a
thin layer of carbonaceous material was formed at the
pushing flight due to the formation of Moffat eddies [13].
Moffat eddies are recirculation or vortices that occur at
sharp corners as shown in Figure 14. When fluid is put in
motion with top driven cavity flow the main circulation is
shown in Figure 14. A secondary circulation is set up in the
stationary corners of the channel, creating a low velocity
helical eddy that is outside the high velocity flows of the
main part of the channel.
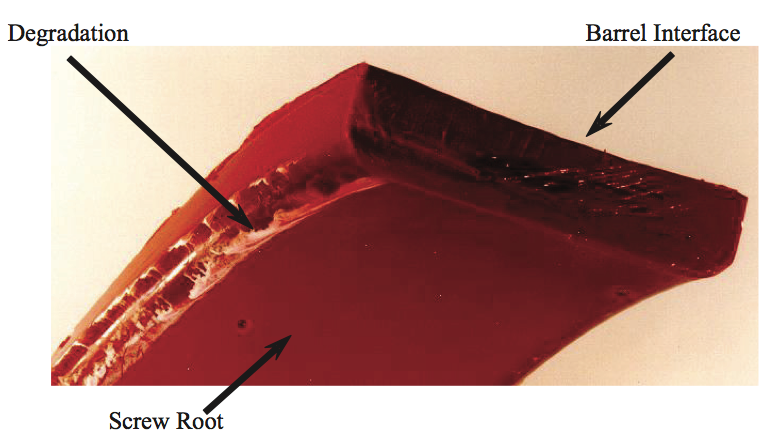
Figure 13. Photograph of degradation at the pushing flight for a screw running LLDPE resin [12].
The Moffat eddies that created the degraded resin
occurred because the flight radii were too small for the depth of the channel. If the flight radii would have been
larger, the Moffat eddies would not have occurred and thus
carbon deposits would not have formed.
The Society of the Plastics Industry, Inc. (SPI)
guidelines state [14] “unless otherwise specified the root
radius will not be less than 1/2 of the flight depth up to 25
mm radius.” Many screws are often designed, however,
with flight radii that are very small and approach values
that are between 10 and 20% of the channel depth.
Previous research [12] has indicated that the SPI guideline
as a minimum is appropriate for many resins. But for
thermally sensitive resins, radii up to 2.5 times the depth
are optimal. Flight radii sizes are shown in Figure 15.
When a new screw with radii equal to the depth of the
channel was built and installed into the blown film line, the
black specks were essentially eliminated.
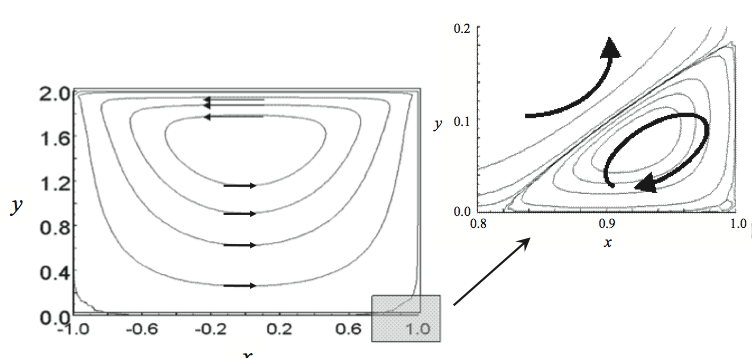
Figure 14. Two dimensional flows in a screw channel with an H/W = 1 (channel depth / channel width). The arrows show the recirculation flows. The shaded area in the lower right corner is expanded to show the Moffat eddy [1].
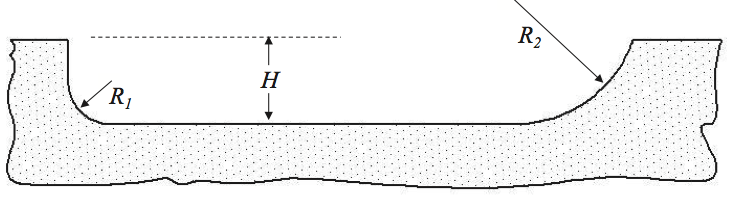
Figure 15. Schematic of small (R1) and large (R2) flight radii.
Filler Agglomerates
Some specialty films are produced using
masterbatches with high levels of mineral fillers. The filler
materials must be compounded with a properly designed
process such that fillers are not agglomerated prior to
dispersion into the base resin. If agglomerates are produced
and contained in the masterbatch, then they are essentially
impossible to disperse in the filming process, leading to
optical defects in the film. For example, a compounding
operation for making a specialty resin from a high impact polystyrene (HIPS) resin and specialty filler chemical was
not designed properly. Here the filler chemical was
partially agglomerated prior to the melting process in a
twin-screw extruder. As shown in Figure 16, the resin was
colored black and the filler chemical was white. These
white agglomerates could not be eliminated in the final
plasticating process (injection molding in this case) and
created defects in the product. The goal for this type of
application is to produce masterbatches that are free of
filler agglomerates since the final film making extrusion
process is incapable of dispersing them.
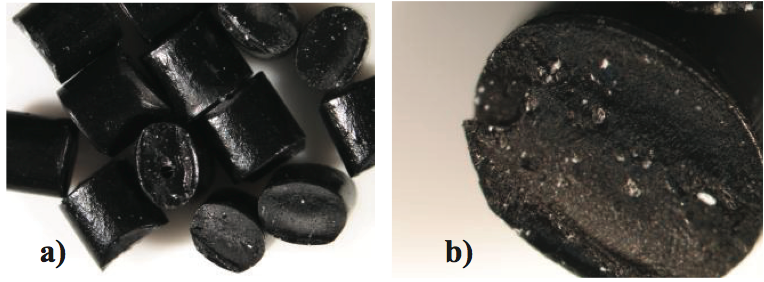
Figure 16. Photographs of specialty HIPS resin pellets made using a poorly designed process. The white specks are filler agglomerates: a) 1x magnification, and b) 4x magnification.
Discussion
Gel defects are common in PE film products, and they
can originate from many different sources, causing a
reduction in the product quality and sometimes stopping
production. Gel types, identification protocols, and
mitigation strategies were presented in this paper.
Mitigating or eliminating gels quickly via the best
technical solution will reduce costs to the plant and
maximize profits.
The equipment and techniques required to diagnose
properly many of the gel types can be expensive and
require highly trained people. Many small converters will
not be able to afford the development of these types of
capabilities. Most resin suppliers, however, have the
capabilities and are willing to aid customers on the
identification and mitigation of the gels.
Summary
This paper describes the different type of gels that are
likely to occur in polyolefin film products, techniques for
identifying the gel type, and technical solutions to mitigate
them from single-screw extrusion processes.
References
1. G.A. Campbell and M.A. Spalding, “Analyzing and
Troubleshooting Single-Screw Extruders,” Hanser,
Munich, 2013.
2. T.I. Butler, “Gel Troubleshooting,” in “Film Extrusion
Manual,” Chapter 19, Edited by T.I. Butler TAPPI
Press, Atlanta, GA, 2005.
3. J. Scheirs, “Compositional and Failure Analysis of
Polymers,” Wiley, New York, 2000.
4. S. Kodjie, S. Guerra, and N. Savargaonkar, 2012
Microscopy and Microanalysis Proceedings, 715
(2012).
5. E.I. Garcia-Meitin, G. Bar, J. Blackson, and D.
Reuschle, Microscopy and Microanalysis, 14 (Suppl.
2), 1380 (2008).
6. J. Blackson, E. Garcia-Meitin, and M. Darus.
Microscopy and Microanalysis, 13 (Suppl. 02), 1062
(2007).
7. D.A. Hemsley, “Applied Polymer Light Microscopy,”
Elsevier Applied Science, London, 1989.
8. K.S. Hyun, M.A. Spalding, and J. Powers, SPEANTEC
Tech. Papers, 41, 293 (1995).
9. M.A. Spalding, SPE-ANTEC Tech. Papers, 50, 329
(2004).
10. K.S. Hyun and M.A. Spalding, “Troubleshooting TPU
Resin Extrusion Rate Limitations Due to Solids in the
Discharge,” SPE-ANTEC Tech. Papers, 58 (2012).
11. B.H. Maddock, SPE J., 15, 383 (1959).
12. M.A. Spalding, J. Dooley, and K.S. Hyun, SPEANTEC
Tech. Papers, 45, 190 (1999).
13. H.K. Moffat, J. Fluid Mech. 18, 1 (1964).
14. “Recommended Dimensional Guideline for Single
Screws,” The Society of the Plastics Industry, Inc.
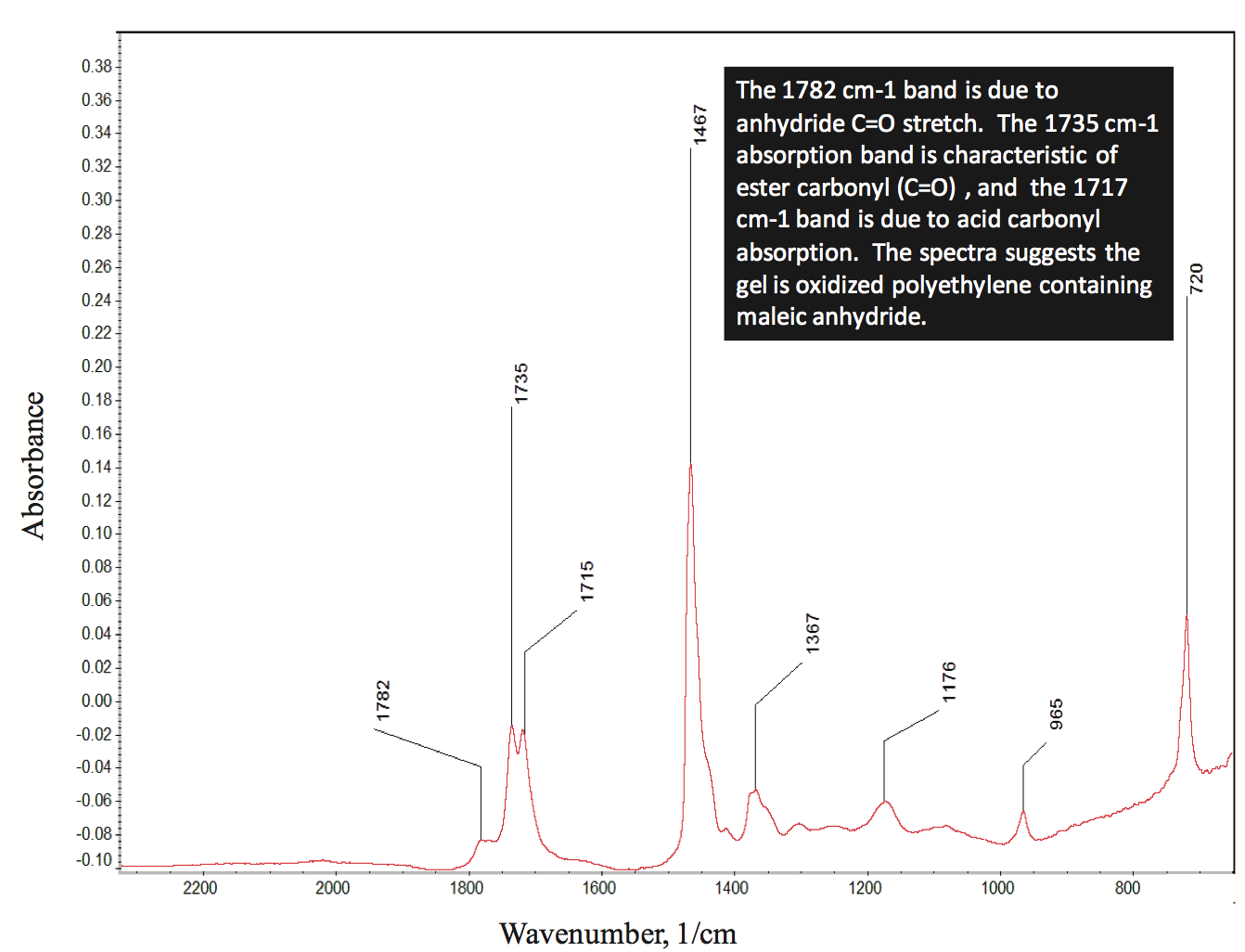
Figure 3. The micro-infrared spectrum of gel shown in Figure 2. The spectrum suggests it is an oxidized polyethylene gel containing maleic anhydride.
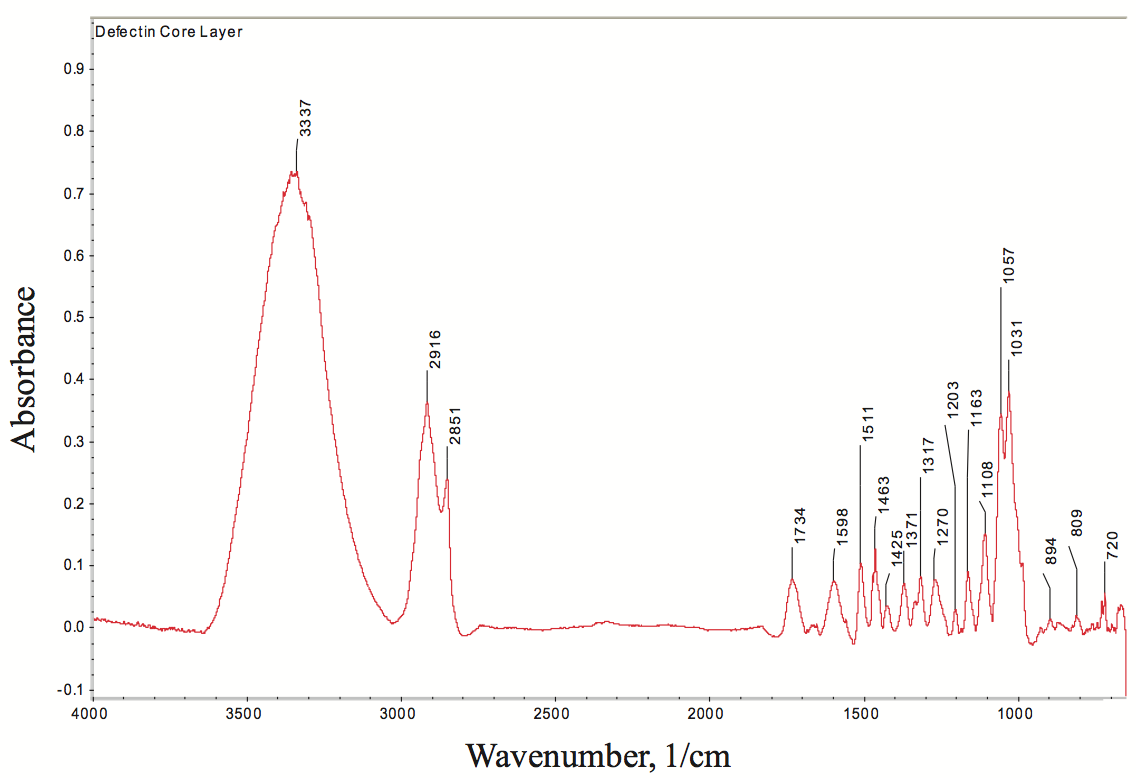
Figure 9. FTIR spectrum of defect in a polyolefin film. The spectrum indicates that the gel is cellulosic fiber and degraded PE resin.
Return to
Paper of the Month.