Applying Basic Solids Conveying Measurements to Design and Operation of Single Screw Extruders¶
G. A. Kruder and Robert E. Nunn - HPM Corporation, Mount Gilead, OH 43338
IntroductionSince the 1975 ANTEC paper by Kruder (1) titled "Fundamental Mechanisms Related To Single Screw Extruder Feeding", other investigators (2, 3) have made considerable use from this paper of solids conveying data obtained with equipment shown in Figure 1. In recent years, we have continued to use this equipment extensively to study crammer-feeding of difficult to feed materials, after adding various spiral and agitator configurations to the rotating shaft in the conical hopper above the screw and barrel. During the past few months, we returned to the original configuration, as shown in Figure 1, to obtain solids conveying data for additional polymer feed stocks with a smooth barrel and also with a longitudinally grooved barrel.
We were especially interested in whether a 'Wider variety of polymer feed stocks would show major deviations from our earlier data. As reported in Kruder's (1) earlier paper, output as a function of discharge pressure for our short 2.1 turn square-pitch extruder screw was virtually identical for three of the four polymer feed stocks studied. This is illustrated graphically in Figure 2.
Our present paper includes data for a variety of polymers, data for various feed particle sizes, and data for one polymer with different additives and moderately different temperature conditions. As would be expected, the grooved barrel increased the output for all feed stocks and completely overcame the output deficiency observed for lubricated feed stocks with the smooth barrel. Based on the data obtained, we have gained additional insights into extruder performance, as discussed below.
Basic Considerations in Obtaining Solids Conveying DataThe first few feed flights in an extruder can be extremely important, since output obviously cannot exceed the solids conveying capacity of these first few feed flights. Furthermore, uniformity of pressure development in these first few feed flights may be important because pressure is believed to rise exponentially with axial length (3). In the first few feed flights solids conveying frequently involves cold pellets being transported at low pressures against a relatively cold or slightly warm wall with the rate very sensitive to pressure. Many extruder manufactures find it desirable to provide a water cooled feed housing directly below the feed hopper, and it is not unusual for the inside barrel surface downstream of the feed hopper to remain well below the polymer melting temperature for at least one or two turns, even when barrel zone # 1 is heated. There need be no significant heat buildup in the first few feed flights unless pressure buildup is rapid.
Other investigators (4) reported that the rate of heat generation at the barrel wall is directly proportional to the local pressure, the coefficient of friction against the barrel, and the rotational speed of the screw. At low pressures (below 4 psi) our measurements have not significantly exceeded the 0.1 to 1. 0 horsepower range for frictional heat generation with our 2.1 turn 4.5" diameter 1.0" deep screw for any pelletized feed stock, even at 90 RPM. The fresh cold polymer moving forward in the extruder absorbed most of the frictional heat genera!ed without causing a major rise in barrel wall temperature.
Solids conveying measurements in this paper are useful when applied to the first few turns of extruders operating in the manner herein described. Downstream of the region
where solids frictional forces control conveying, the barrel surface gets hot, and the viscous drag mechanism for solids conveying contributed by Chung (5) is believed to apply. Data obtained in this paper are in close agreement with the theory of Darnell and Mol (6) for zero discharge pressure. However, even at very slight back pressures the Darnell and Mol theory often becomes impractical for calculation because coefficients of friction against the screw and barrel surfaces can be pressure dependent and difficult to determine. For this reason we consider it much more practical to actually measure solids conveying throughput as a function of back pressure with our apparatus rather than attempt to calculate it. Using the pressure measurement at the end of our 2.1 turn screw and an estimate of initial pressure at the start of the screw, we can approximate the pressure rise for other screw lengths since Darnell and Mol (6) pointed out that ln P
2/ P
1 is directly proportional to length.
The basic solids conveying equation of Darnell and Mol, given below, is easy to use when there is no pressure buildup.
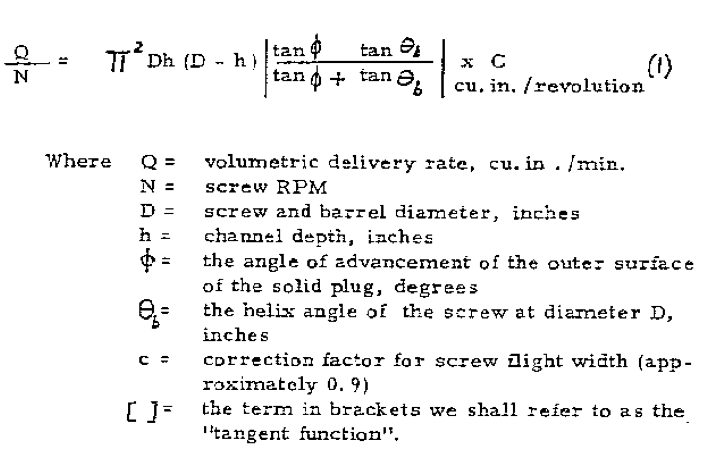
To calculate volumetric output per revolution every item in the equation can be determined from screw geometry except tan theta. The angle theta can be calculated from rather complex
force balance equations, which lead to simple solutions only for a few special situations. If we assume that our 1.0" deep 2.1 turn screw helix, aided by gravity, requires virtually no "push" from the screw flight to move feed down the short tubular barrel segment at zero discharge pressure, then the output should closely approximate frictionless screw under the Darnell and Mol theory. For this situation the "tangent function" equals 0.29, and the output at zero discharge pressure calculated for our 1.0" deep screw is 40.66 cu.in.per revolution.
In Kruder's earlier paper (1) measurements of output at zero discharge pressure agreed closely with the Darnell and Mol equation. For free-flowing feed stocks studied for our present paper this was true also, using a smooth barrel, except where highly lubricated feedstocks yielded additional output due to gravity.
Calculation of the angle theta for discharge pressures above zero can be extremely complex. Tadmor and Klein (7) reported the following equation which becomes identical to an equation
derived by Darnell and Mol (6) when two simplifying assumptions are made.
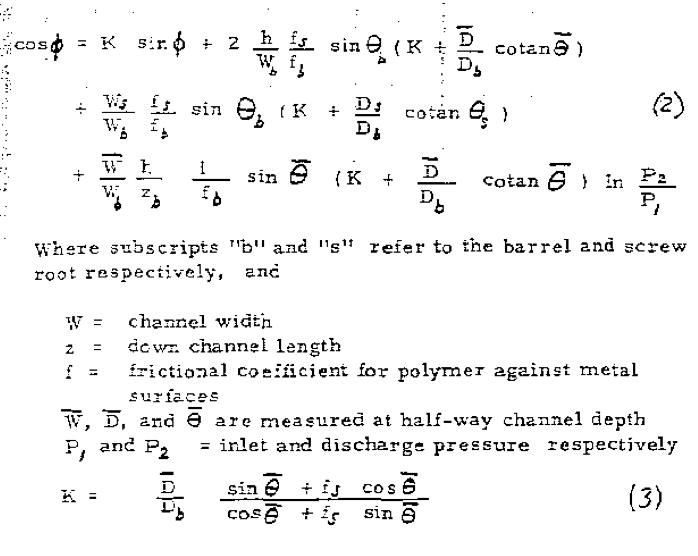
Everything in Equations (2) and (3) can be calculated from screw geometry except for f
s, f
theta, P
1, and P
2. Recent studies (3) dealing with measurement of friction coefficients indicate that they can vary with pressure, temperature, and screw speed. Consequently, Equation (2) may be extremely difficult to apply with a high degree of reliability. On the other hand, direct measurement of output as a function of P
2 can be made readily with our apparatus, shown in Figure 1. Then the angle theta can be simply calculated from Equation (1) if desired, as shown graphically in Figure 3 for our 1" deep 4.5" diameter screw. Our data would indicate that the angle of advancement of the solid plug typically shifted all the way from 72° to only about 10° when discharge pressure rose from zero to only about 2 psi. Therefore, our direct measurements of output as a function of pressure would appear to be much more dependable than coefficient of friction measurements inserted in Equation (2) for predicting extruder feeding performance.
Describing of Apparatus, Feed Stocks, and Test ConsiderationsThe equipment for solids conveying studies, shown in Figure 1, consisted of a 2.1 turn 1.0" deep square-pitch 4.5" diameter screw in a short vertical barrel. Except for special test situations the conical feed hopper was kept filled at all times by an automatic filling device. The discharge pressure was regulated by adjusting the height of the hydraulic jack, and the pressure was measured by the hydraulic pressure developed in the jack. For low pressure ranges (below 25 psi., equivalent to about 430 lbs. force against the jack ) a 500 lb. capacity Martin-Decker load cell was inserted over the jack for more sensitive load measurements with the smooth barrel. The following data were recorded:

Extensive data tables too lengthy for inclusion in this paper were accumulated, similar to tabulations in Kruder's (1) earlier paper. All output versus pressure data for all feed stocks were plotted graphically, as for example in Figure 4. However, space is not available to present all of the graphs in this paper; so the graphs were used to reconstruct data in condensed form for Table 1. Graphs can be re-drawn with reasonable accuracy from values in Table 1.
Feed stocks included in the test program are described in Table II. Bulk density was determined for all feed stocks, and relative pellet size was approximated by counting the number of pellets per gram. The angle of repose for a pile of feed particles on a cylindrical disk also was measured, and lubricated feed stocks exhibited a low angle, as would be expected. Using the apparatus shown in Figure 1, lubricated feed stocks required a much narrower discharge gap (between he bottom of the barrel and the restraining plate above the jack). At 60 RPM 1 all non-lubricated feed stocks required a gap setting above 0.4" to maintain 10 psi. back pressure; whereas the high slip PE 125 EQ and the lubricated PS beads had to be set below 0.2" to achieve equivalent back pressure.
ABS powder feeding studies have utilized various spiral and agitator configurations mounted on the rotor directly above the screw to avoid bridging and to provide feed compaction.
Only the smooth barrel was used for ABS powder feeding. All other feed stocks were run on both the smooth and grooved barrels. Both barrels were identical except for the grooves. Equally spaced around the inside barrel surface were ten sharp-edge longitudinal grooves 0.156" deep and 0. 825" wide at the bottom. Both barrels were equipped with two thermocouples located within 0. 3" of the inside wall. The # 1 thermocouple was positioned at 1.22 L/D and the # 2 thermocouple at 1.92 L/D, relative to the 2.1 L/D 4.5" diameter square-pitch 1.0" deep screw with 0.375" flight width.
In collecting data with the smooth barrel, most pellet feed stocks did not heat up the barrel wall thermocouples beyond the range of 70° to 100° F. except where discharge pressure was
set above 10 psi. Then wall temperature rose rapidly for some feed stocks, while DC drive amperage also rose significantly over the no load value. However, over the limited range of test conditions studied with pellet feed stocks and the smooth barrel, temperatures appeared to have little effect on the graphs of discharge pressure versus output. Figure 5 includes PE 125 NA data for cold pellets (70° - 74 F°.) and cold wall (70° - 90° F. ), versus warm pellets (up to 142° F.) and warm wall (up to 176° F.), versus cold pellets and warm wall. Despite these temperature variations all the data at 60 RPM screw speed fall virtually on the same curve. Of course, at still higher temperatures where pellets begin melting at the wall surface, we would expect major deviations from the Figure 5 curve. Highly lubricated pellets also may exhibit greater output deviations with temperature.
In another experimental solid conveying study by Mizoguehi (8) it also was evident that moderate changes in barrel temperature (below 80° C. with a neutral screw) exhibited little affect on output for most feed stocks. From design of Mizoguehi's apparatus it appears likely that his data at "no back pressure" actually may have been at slight back pressure. Most of his data cover a much higher pressure range up to about 700 psi. for a 5 L/D extruder with hotter screw and barrel temperatures. However, his graphs show an "output floor" (similar to our findings)
beyond which higher pressures only slightly reduce output even though high pressure would generate considerable frictional heat. Consequently, it seems likely that the output versus pressure curves obtained in our study would not have been drastically different even if we had allowed the barrel temperatures to rise a little more.
The cooling coil around our barrel was not always adequate to maintain the barrel temperature within desired control limits, so we sequenced test conditions to minimize the problem. Typically, cold pellets at low pressure served to cool a warm wall; whereas high pressure caused wall temperature to rise. Consequently, we would follow a high pressure data point with a low point whenever necessary to keep down the barrel temperature.
Results of Solids Conveying Studies with Smooth BarrelTable I presents a condensation of all data obtained with an unheated barrel relating output to discharge pressure for each feed stock except ABS powder. Graphs like Fig. 4 can be easily plotted from these data, but space permits presentation of only a few similar graphs of special interest.
Figure 4 shows that PE 125 low density polyethylene output as a function of discharge pressure is drastically influenced by slip agent content and to a lesser degree by screw RPM using a smooth barrel. The no additive material PE 125 NA at 60 and 90 RPM yielded outputs significantly above the values plotted in Figure 2 from Kruder's earlier paper (1), whereas the high slip PE 125 EQ yielded much poorer outputs. Most other feed stocks in our new study also showed significant deviations from the values plotted in Figure 2. Nevertheless, the fact that three out of four feed stocks covered in the earlier study closely fit the same curve in Figure 2 is not without some significance.
Like the earlier study, all results confirmed the validity of the basic Darnell and Mol equation as applied to a frictionless screw operating at zero discharge pressure. The data in Table I at zero discharge pressure is subject to a slight gravity flow error for most feed stocks and a more serious error for the highly lubricated feed stocks, namely the high slip PE 125 EQ and the lubricated Cosden PS 825 beads. For these latter two feed stocks at zero discharge pressure and zero screw RPM the flow reached 800 lbs. per hour and more, but flow became zero when even the slightest observed pressure was applied. Except for the high slip PE 125 EQ the gravity flow error was Virtually completely eliminated at go RPM for zero discharge pressure and was considered negligible at any higher pressure for all materials and screw speeds. Note the exceptional agreement between calculated and measured output at 90 RPM and zero discharge pressure, except for PE l25 EQ, as shown below
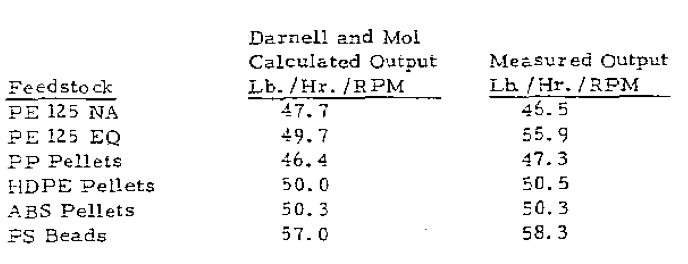
Like the earLier study, every feed stock exhibited a drastic drop in output under even slight back pressures (below 1 psi.). Also, under moderate back pressures (2 to 10 psi) the slope of the output versus back pressure curve always shifted drastically from nearly vertical toward the horizontal. As observed from Table I, all feed stocks came within about 10% of leveling of in output between the 10 psi and 15 psi data points at both 60 and 90 RPM except for the high slip PE 125 EQ and the HDPE. These results conform basically to the characteristics of output versus back pressure curves from Kruder's (1) earlier study. If we choose to call the average output between 10 psi and 15 psi. the "output floors" (whenever the 15 psi output is at least 90% of the 10 psi. output), we can say that the "output floors" for most pellets feed stocks studied to date show only moderate differences, and also only a moderate shift with screw RPM. 'The major exceptions measured to date have been two types of HDPE and the high slip PE 126 EQ, which failed to develop well defined "output floors".
APPLYING SOLIDS CONVEYING DATA TO EXTRUDER DESIGN AND OPERATIONThese exceptions do not necessarily signify an extrusion feeding problem if screw design and operating conditions are well suited to the application. As an example, note the Figure 6 graph, which shows output versus discharge pressure for Hoechst HDPE, Type GM 5010 T2N. Data at all screw speeds studied closely fit a single curve, but the output does not level of sufficiently to identify an "output floor". Nevertheless, this HDPE can develop 5 psi. in just 2.1 screw turns while conveying 1400 lbs. per hour at 100 RPM. At 2 psi. the conveying capacity would rise to 2,000 lbs. per hour. At double the solids feeding length we typically could expect about a 10-fold rise in Pressure development so even 2 psi. at 2.1 L/D is a reasonably good start if the feed length is adequate.
Horsepower consumption in the first 2.1 tuns for dry solids conveying against an unheated barrel is almost negligible unless pressure buildup is rapid. In Kruder's (1) earlier paper the
net horsepower never exceeded 2.0 at 5 psi. but became much more significant at high pressures. Consequently, high pressure would be expected to add frictional heat rapidly and shorten the distance before viscous drag (5) dominates solids conveying.
Again examining Figure 6, if the screw design and operating conditions downstream restricted output sufficiently to build up 20 psi. at 2.1 L/D, then the feed section would deliver only 5 lbs./hr/RPM while consuming very little horsepower to generate frictional heat at the barrel wall. By contrast, if the downstream screw design and operation restricted PE 125 NA output to twice this much, say to 10 lb/hr./RPM at 60 RPM, Figure 5 shows that the pressure would extrapolate to a very high range. Then horsepower consumption in the first 2.1 turn would become very significant resulting in rapid frictional heat buildup and an early start to melting, all of which might partially overcome the downstream deficiency. In other words, we might expect PE 125 NA to feed adequately over a broader range of extruder screw designs and operating conditions.
We are not yet ready to suggest any universal guidelines for screw design based on these pressure versus output curves. However, it would seem prudent to select a feed depth where the extruder would operate within the 2 psi. to 10 psi. range at the 2.1 L/D location at which our data was taken. In this region the output is reasonably compliant as a function of pressure to downstream extruder performance, but localized frictional heat buildup is unlikely to be high. We observed that pressure development always was quite steady up to about 5 psi. and became progressively more variable for higher pressures at the 2.1 L/D location. In special situations, however, where heat sensitivity is not a problem and screw length is a limiting factor, such as for the short first stage of a 24 L/D two stage vented screw, it may be advantageous with some feed stocks to design for rapid pressure buildup to initiate melting faster and increase output. For extremely rapid pressure buildup a grooved barrel can be considered.
Results of Solids Conveying Studies with Grooves Barrel
As evident in Figure 4 for high slip PE 125 EQ, some feed stocks (particularly with slip agents or other greasy or waxy additives} never reach an "output floor" below which they can be counted on to begin rapid pressure development. For such materials longitudinal grooves in the barrel for a short distance in the feed section can be very beneficial, as reported by Duska. (9) at the 33rd. ANTEC in 1975. Also, longitudinal grooves have other applicators and are widely used in Europe for low melt index HDPE, particularly for blow molding extruders.
Our results comparing output versus pressure for longitudinally grooved barrel are shown in Table I, alongside results for our smooth barrel. Design and use of our grooved barrel was guided by research information published by the IKV(10), which has studied extensively applications for grooved barrels. All of our data with the grooved barrel for every feed stock showed distinctly higher outputs than with the smooth barrel under equivalent back pressure. The high slip PE 125 EQ actually yielded superior output by the no additive PE 125 NA with the grooved barrel. However, all pellet feed stocks were fairly close together in output, especially at high pressure in the range of 50 psi. Note in Table I that the lowest output was 39.2 and the highest output 49.9 lb. /hr. /RPM among ali pellet feed stocks at 50 psi. back pressure.
Since the grooves tend to lock compacted pellets against the barrel wall so as to prevent rotation, each revolution of the screw theoretically should transport the volume of feed in the barrel for one turn of the screw. For our 4.5" diameter 1.0" deep square-pitch screw this volume is 50.1 cu. in. per revolution ( including groove cavities). Converting to lbs./hr. /RPM using bulk density data from Table II, the calculated versus measured outputs (from Table 1) for all pelletized feed stocks at zero back pressure are listed below.
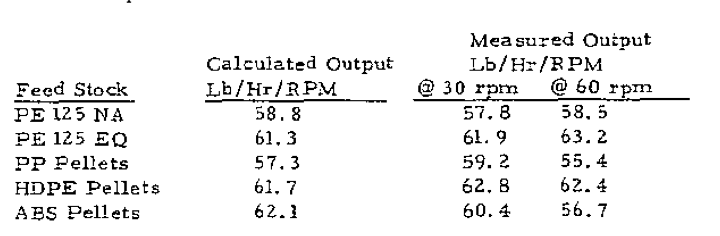
In general, the measured versus calculated values were in close agreement at zero back pressure. However, Table I shows that output dropped substantially at very low back pressure (e.g. 2 psi) and arrived at a well-defined "output floor" between 5 and 10 psi. However, for pellet feed stocks in these studies output never dropped more than 33% from maximum, even at 50 psi.
One might ask whether a grooved feed housing is the answer to every problem, and the answer is "NO" . The pressure stability for a grooved feed section is sometimes difficult to maintain, and we find that use of a well designed melt metering section to control output uniformity through the die can be superior. Furthermore, the grooved feed housing may be capable of delivering more feed than the downstream sections of the extruder can accommodate, resulting in equipment breakage unless the total design and operating conditions are well matched. Fortunately, rapid pressure buildup results in rapid frictional heat generation, which eventually lowers the transport efficiency of the axial grooves. Therefore, catastrophic experiences with axial grooves are not likely if the grooved section is kept short and not too cold. Nevertheless, for uniform gauge control (as needed for sheet, film, pipe, and profiles) grooved feed housings should not be considered as a substitute for the pumping action of the screw melt metering section. Nevertheless, to handle special situations, especially for compounding lubricated feed stocks, axial grooved feed housings can be used to advantage.
When developing high back pressure with a grooved feed housing, melting can initiate primarily on the pushing side of the feed flights, as shown in Figure 7 for the ABS pellets. Except for fused pellets against the pushing side of the screw flight and in the adjacent barrel grooves all of the remaining pellets discharged cold and free-flowing at an average temperature at 80° F. Under test conditions there never were pellets stuck to the screw flights. Our test barrel for the 2.1 turn screw was 3.1 L/D in length, which accounts for the length of the unwrapped spiral shown in Figure 7. This spiral shows that pellet fusion at the screw face began within the first turn of the screw under short-term operation at 30 RPM and 73 psi back pressure (over 1100 lbs. force developed against the jack). The initiation of melting for a grooved barrel at high back pressure can be quite different than for a smooth barrel.
RESULTS OF POWDER FEEDING STUDIESAs shown in Table II, all pellet feed stocks ranged in bulk density from about 33 to 36 lb. per cu. ft.; whereas the ABS power bulk density was only 20.8 lb. per cu. ft. Under 1 psi. pressure it compacted to 25.5 lb. per. cu. ft., and under 5 psi. it compacted to 26.4lb. per cu. ft. This ABS powder was not sufficiently free-flowing for gravity feed; so a one turn compacting spiral was added in the conical section of the feed hopper directly above the 2.1 turn 1.0" deep screw.
Output versus back pressure curves obtained for 30 RPM and 60 RPM screw speeds are shown in Figure 8, operating with a smooth barrel. The 30 RPM data curve was significantly above the 60 RPM data curve in output. Both data curves showed a reasonably well-defined "output floor", no worse than typical for pellet feeds except for the reduction expected due to density difference alone. We know from other feeding tests with similar ABS powders that longer compacting spirals above the screw and use of even deeper feed screws can completely overcome most feeding limitations. In fact for ABS powder, we have reached outputs above 20 lb./hr. /RPM at 20 psi. back pressure with 2.1 turn 4.5" diameter screws at 100 RPM, equivalent to 2000 lb. per hr.
Most powders can be extruded very satisfactorily in smooth barrels if they are prevented from bridging in the feed hopper and if they can be adequately compacted in the feed section except where poor frictional characteristics prevent successful feeding (as for example because of greasy or waxy additives). Some powders are sufficiently free-flowing and dense for good feeding without a crammer-feeder, but others require force-feeding for good extruder output.
MISCELLANEOUS OBSERVATIONSDuring the course of this investigation we observed that output measurements versus discharge pressure were highly reproduceable for most feed stocks, especially for the 60 and 90 RPM measurements. For example, with the PE 125 NA feed stock we made three measurements of output at each of six discharge pressure settings (above zero) at 60 RPM and also at about 90 RPM. Among these 36 readings no reading deviated more than ±0.74 % from the average of the three measurements. Most other feed stocks showed similar output stability. However, the ABS and polypropylene pellets and the ABS powder were noticeably inferior in output stability. Pressure stability for all feed stocks deteriorated with increasing back pressure above 5 psi.
We also observed that output stability for the pelletized feed stocks did not appear to be affected by level in the feed hopper, which had been a concern to other investigators (3). We carefully measured the output as a function of hopper fullness for two polymers and found no effect. For example, with the PE 125 NA feed stock we allowed the hopper level to gradually change from full to almost empty over a 15 minute period and then operate virtually empty (10-20 lb. hopper load) for the next 15 minutes. Screw speed was maintained at 60 RPM and back pressure at 4.0 psi. Eleven consecutive output measurements were taken by weighing one-minute samples at approximately three minute intervals. The average rate was 20.7 lbs. per min. with a standard deviation of 0.25. All data points were within ± 2.4% of the average. There was no trend in the data toward lower output at low hopper level.
One more observation of special interest was a residual deposition of slip agent on the screw flights and barrel wall from the PE 125 EQ formulation. In re-study of PE 125 NA (no additive) feeding following use for PE 125 EQ (high-slip) , the screw and barrel yielded a gradually shifting output trend for awhile until the surfaces were exposed to considerable no additive polymer. This was best illustrated by the gravity flow output far open discharge at zero RPM. Under such conditions prior to use of our apparatus for the high slip PE 125 EQ, the PE 125 NA gave only 244 lbs per hr. gravity flow. After use for the high slip feed, the apparatus was completely emptied and reloaded with PE 125 NA, which then exhibited 972 lb. per hour gravity flow. Over the next 10 minutes the gravity flow gradually dropped to 480 lbs. per hour. Next, proceeding to a 1.0 psi. pressure setting for a 0.5" discharge gap opening at 50 RPM, the output moved up progressively from 21.2 to over 24 lb/hr/RPM over the next 5 minutes, while the pressure progressively increased 80% over a 12 minute period for the same 0.5" gap opening. However, after a few minutes subsequent operation at about 14 psi back pressure with a smaller discharge gap setting, the metal surfaces were sufficiently "scoured" with the no additive feed to duplicate original performance, even at low back pressure.
If we had not raised the back pressure, it might have taken much longer to "scour" the slip off the screw and barrel wall. This is but one example of how many factors must be taken into consideration in studying solids conveying.
ACKNOWLEDGMENTThe authors gratefully acknowledge the assistance of other EPM Laboratory personnel in equipment preparation and operation to collect test data, especially C. J. Reed, S. T. Takashima, C. W. Wright, and J. L. Wright.
Bibliography- 1. G. Kruder, SPE 33rd ANTEC, VoL 21, p 423-428 (1975)
- 2. I. Klein, SPE 34th ANTEC, Vol. 22, p 444-449 (1976)
- 3. I. Klein and R. Klein, SPE 36th ANTEC, Vol. 24, p 519-524 (1978)
- 4. Z. Tadmor, E. Boyer, and I. Klein, SPE 29th ANTEC, Vol. 17, p 136-140 (1971)
- 5. C. Chung, SPE Journal, VoL 26, p 33-44 (May 19701)
- 6. W. Darnell and E. Mol, SPE Journal, Vol. 12, No. 4, p 20-29 (April 1956)
- 7. Z. Tadmor and I. Klein, "Engineering Principles Of Plasticating Extrusion", Van Nostrand and Reinhold Co., N.Y. (1970)
- 8. M. Mizoguehi, Japan Steel Works Technical News, No. 11, p 1-6 (1975)
- 9. J. Duska, J. Gasior, and A. Pomper, SPE 33rd ANTEC, Vol. 21, p 434 - 438 (1975)
- 10. B. Franzkoch and C. Menges, SPE 36th ANTEC, Vol. 24, p 512-515 (1978)
Return to
Best Papers.
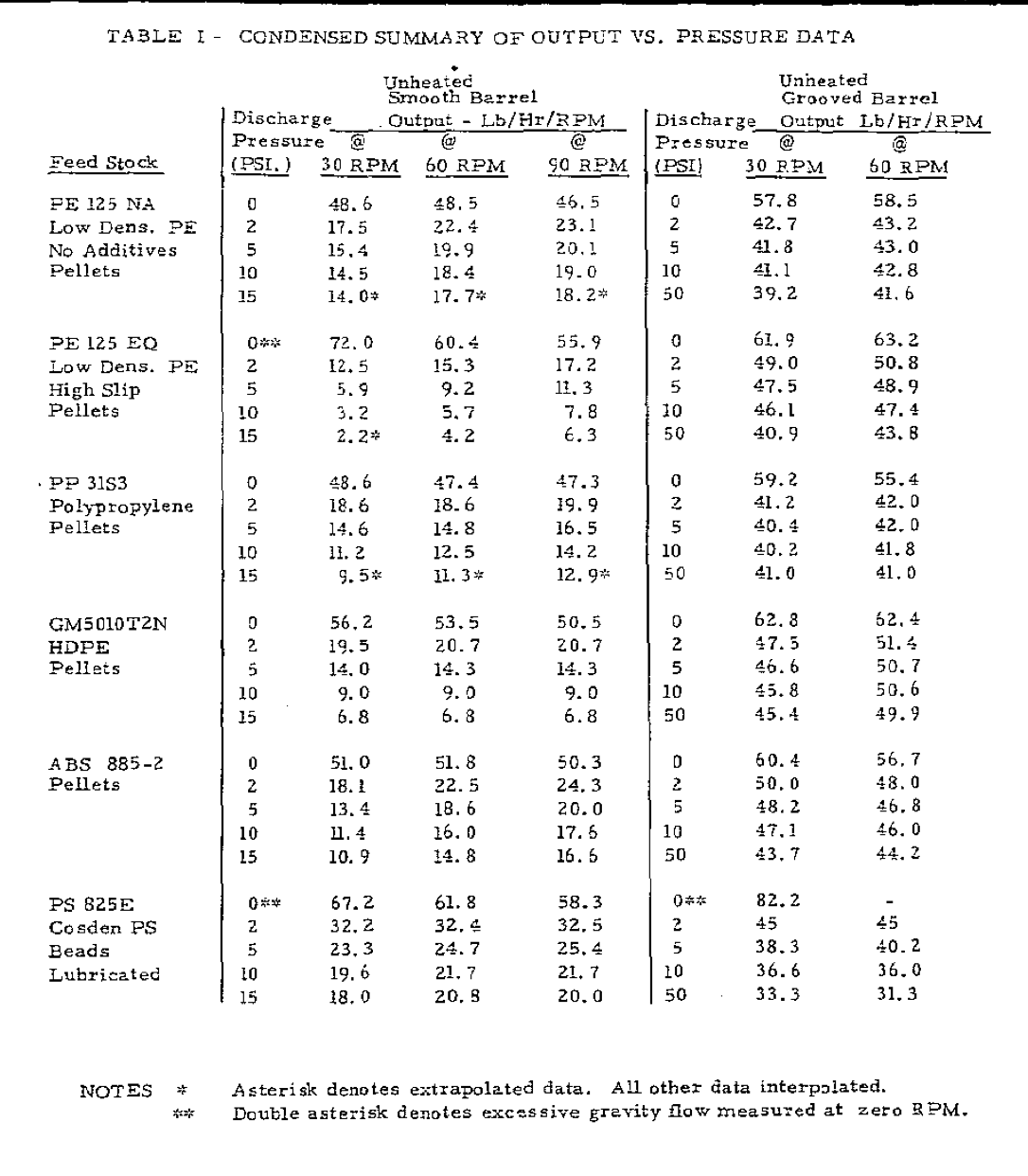
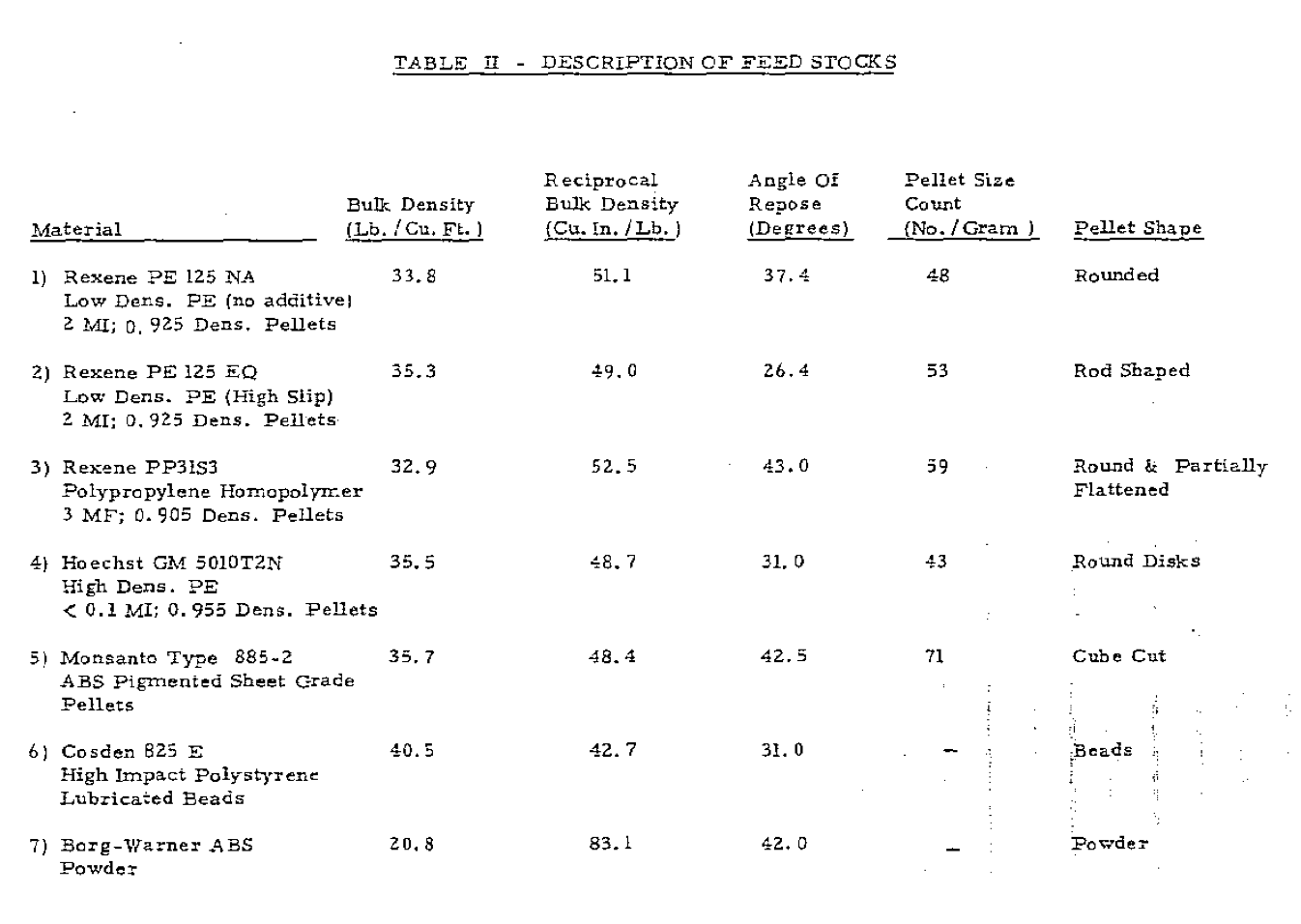
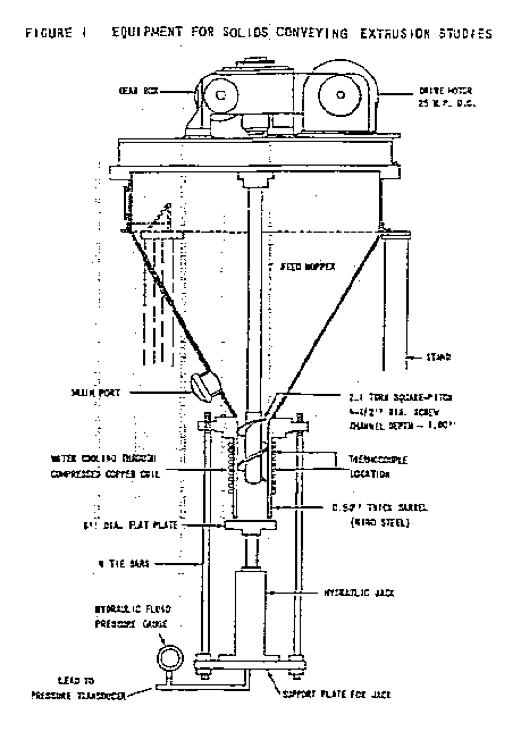
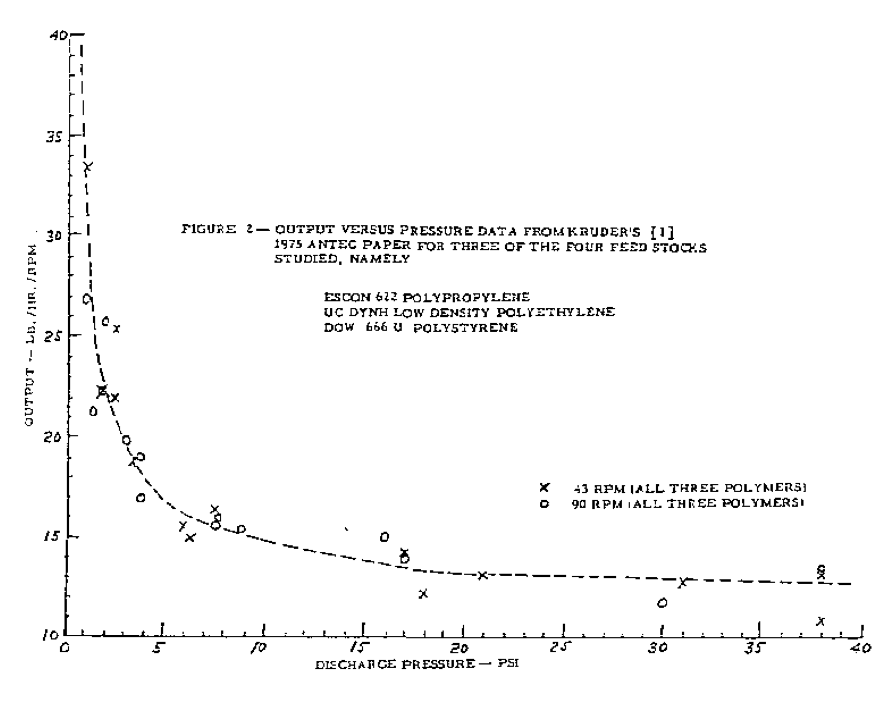
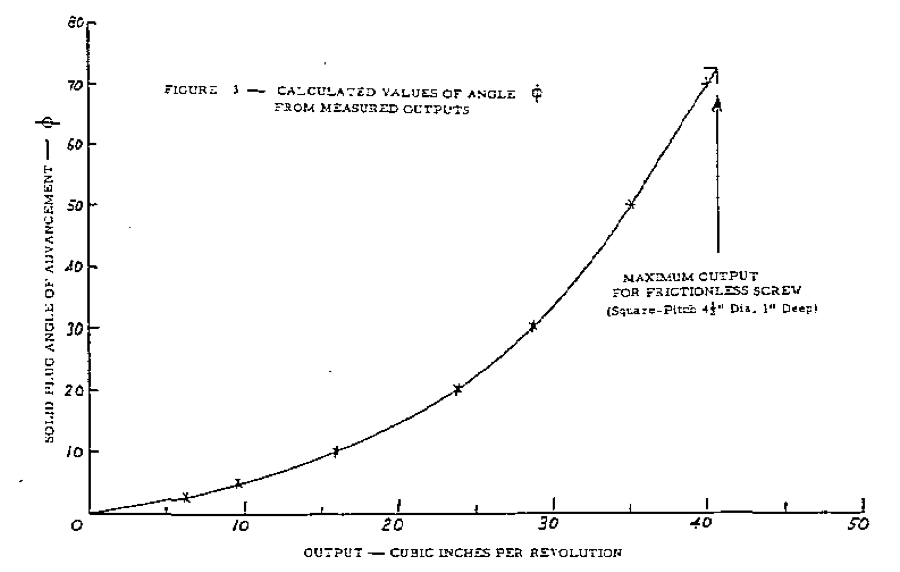
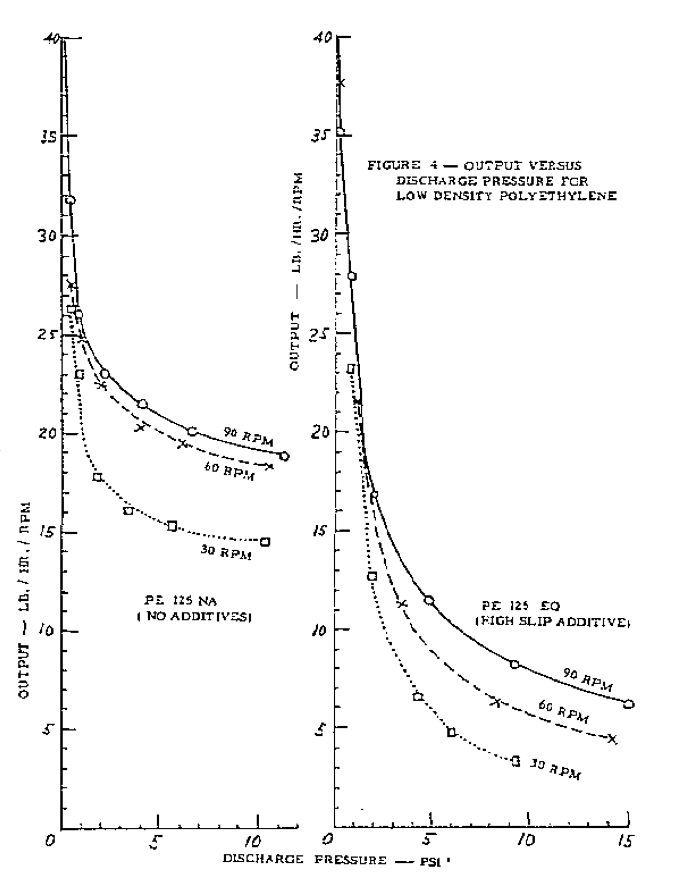
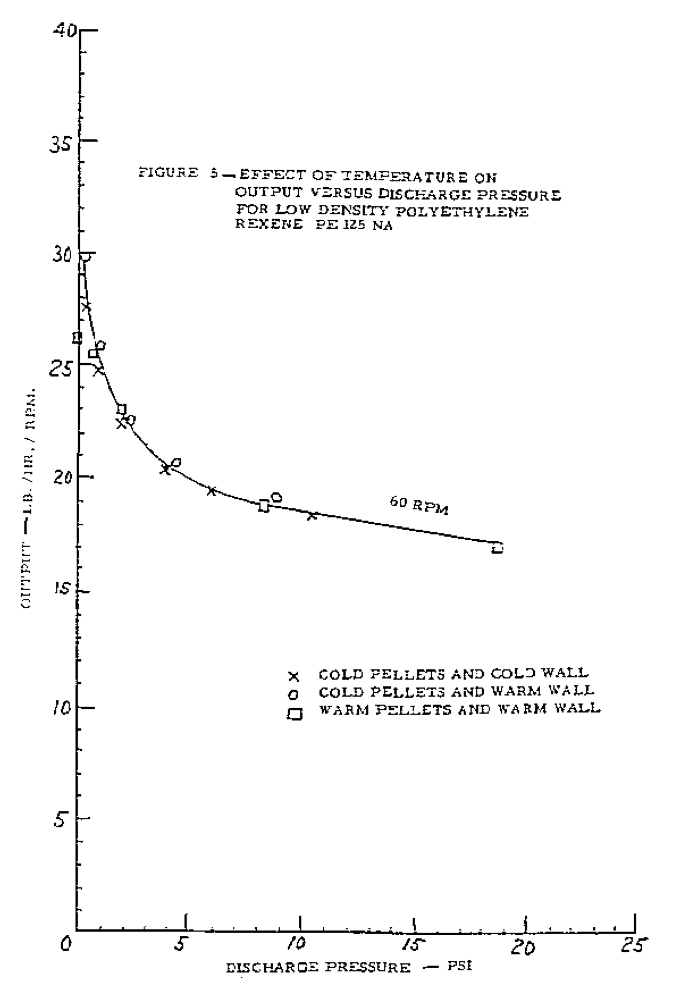
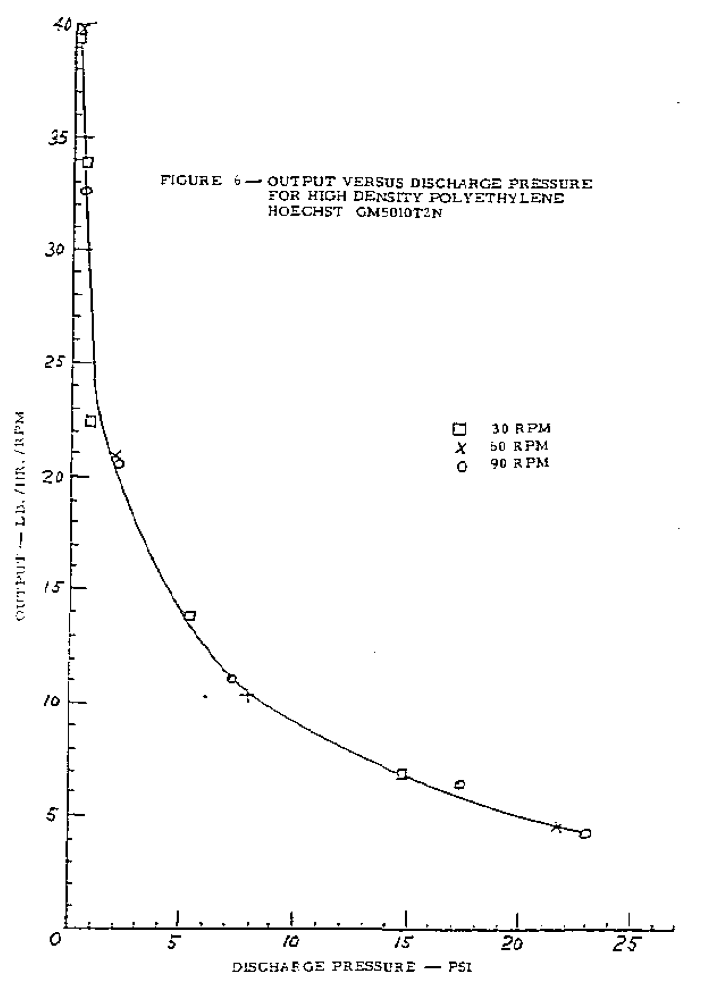
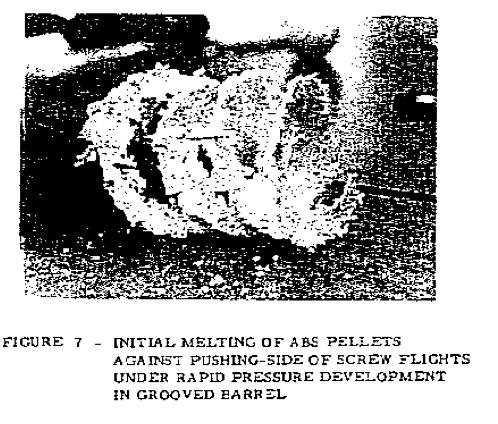
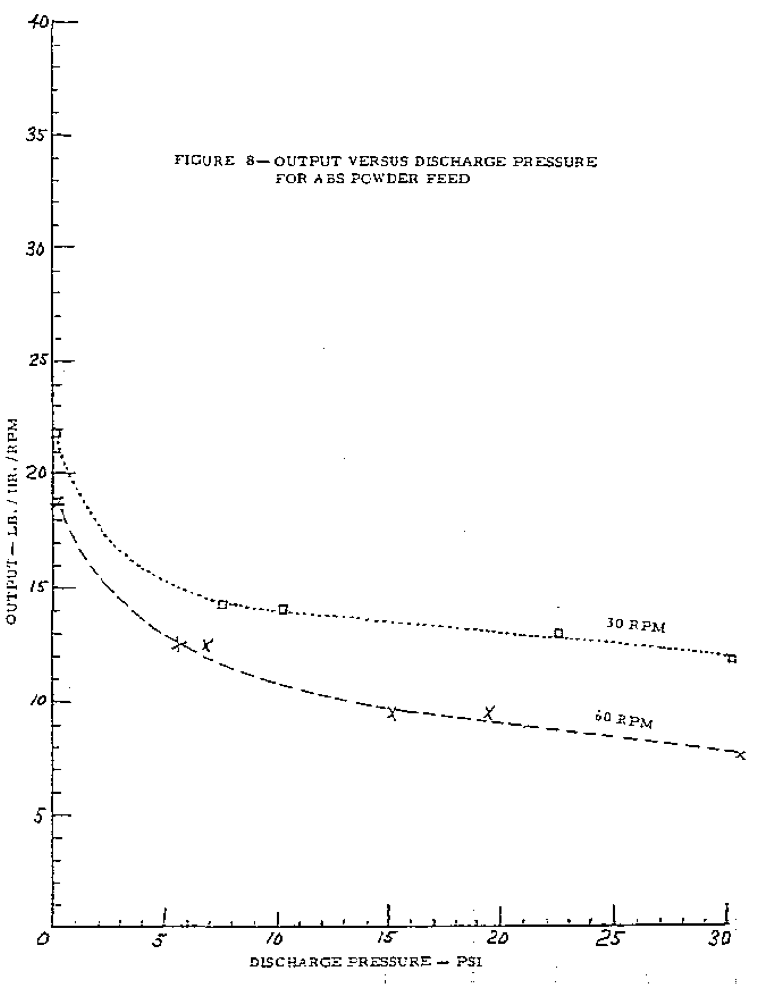