Lateral Stress and Bulk Density of Pet Resin with Re-Cycle¶
Stephen J. Derezinski, Extruder Tech, Inc., Penfield, NY 14526Abstract
Solids conveying depends on the bulk density and
the lateral stress of the feed resin. Laboratory
measurements show that the addition of re-cycle PET
flakes to virgin PET pellet resin feed significantly changes
its lateral stress and bulk density. Data for different ratios
of re-cycle flake to PET pellets are presented. Also, data
for 100% PET pellet feed and 100% PET re-cycle flakes
are given for comparison.
Introduction
Extrusion of PET is widely used for packaging
and films. PET is also readily re-cycled, and the re-cycle
usually is in the form of chips or flakes. When the virgin
PET pellets are then mixed to some proportion with the recycled
PET, solids conveying performance is affected.
Solids conveying has been shown to depend on lateral
stress [1-5] and bulk density [3]. Therefore, the desire to
extrude PET pellets mixed with re-cycle motivated this
laboratory study of the lateral stress and bulk density of
mixtures.
Materials
Virgin PET pellets and re-cycle PET flakes are
shown in Figure 1. The pellets have an average mass of
0.053 gram, and approximate dimensions of 3.2 x 3.2 x 4.2
mm. The pellets and re-cycle were mixed in proportions
of 25%, 50%, and 75% of re-cycle on a total mass basis.
The total mass of each mixture sample was 3 to 4 grams,
and samples were individually weighed for each test. The
mass of each component was measured to 0.01 gram. The
lateral stress and the bulk density of the mixtures were
then measured as a function of primary compressive stress.
Experimental Equipment
Figures 2 shows the test cell from previous work
[2] used to measure the stresses and bulk density of the
different mixtures. Load cells record the axial and lateral
forces and a linear displacement transducer records the
axial strain. The lateral strain is assumed to be zero as in
previous work [4], but some minimal lateral strain does
occur as a result of the deflection of the load cell.
However, it is insignificant in relation to the axial strain.
The data taken with the test cell include the axial
length of the specimen, the axial or primary load force, and
the resulting lateral load force. The dimensions of the test
chamber and the piston displacement measurement provide data to calculate the bulk density of the sample as it is
compressed and reduced in volume. The two load cells
and the dimensions of the test chamber give the needed
data to calculate the axial and lateral stresses developed
during compression.
“Loose” Bulk Density Data
The “loose” bulk densities of the mixtures of
pellets and re-cycle were measured to be a function of the
fraction of PET re-cycle. “Loose bulk density” is defined
as that at minimal or zero stress, and Figure 3 shows the
typical values for the 5 different samples. Pure pellets and
pure re-cycle and proportions of 25%, 50%, and 75% of
recycle mass/total mass are given. The data were obtained
from the test cell before any strain or stress was applied.
Figure 3 shows that the loose bulk density of the pellets is
significantly greater than that of the re-cycle. Figure 3 also
shows that the loose bulk density is reduced in
approximate proportion to the mass of recycle that is
mixed.
Stratification of re-cycle and pellets is likely to
occur in the delivery system of an extruder. Therefore, a
sample datum for 50% ratio of re-cycle mass/total mass is
shown in Figure 3. The loose bulk density is shown to be
nearly the same as for the 50% mixed resin. A slightly
lower bulk density might be expected as the void space in
the pellet portion is only filled with air for the stratified
condition. However, this is shown to not be significant for
the 50% stratified mixture of pellets and re-cycle when
compared to the 50% sample that is mixed.
Bulk Density vs. Primary Stress Data
Data for the bulk density as a function of primary
stress are given in Figure 4. Variation in their value
depends on the primary stress as well as the mixture
proportion. The matter is complicated by the fact that bulk
density of PET pellets will be greater than mixtures at low
primary stresses and less than mixtures at high primary
stresses. The cross-over point of stress is at a primary
stress less than about 15 MPa.
Bulk Density Regression Functions
The curves for bulk density of Figure 4 are
modeled with 6th order polynomials, and they are given
below. The correlation coefficient was greater than 0.999
for all the functions. The range of validity of primary
stress is 0 to 40 MPa. Above 40 MPa error is likely, but a
linear extrapolation for stresses moderately above 40 MPa could extend the useful range if need be. The results are
given in equation form for easy programming to provide
an accurate representation of the density data.
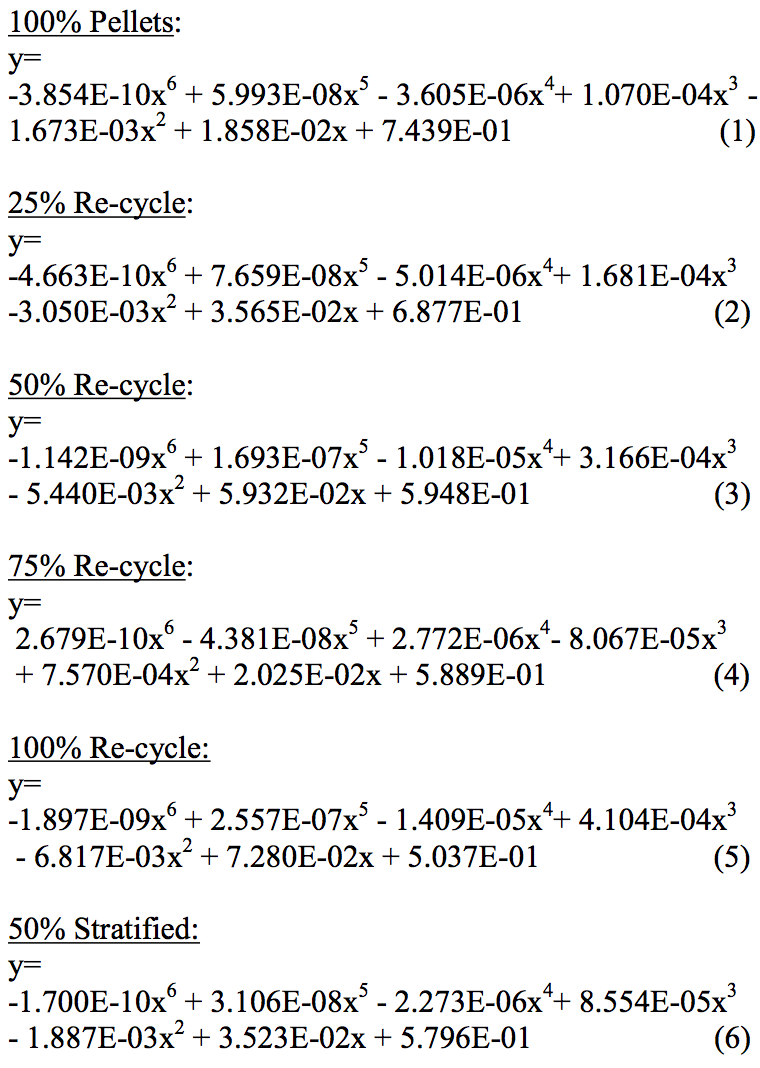
Lateral Stress vs. Primary Stress Data
Figure 5 shows the lateral stress for PET pellets
and re-cycle resin. Data for PET pellets alone (100%
pellets) is one basis for comparison with the mixtures of
pellets and re-cycle. Another basis is the data for 100%
PET re-cycle, and this is shown in Figure 5, also. These
two base line functions appear fairly similar. However, the
lateral stress for the 100% re-cycle is greater than that of
the pellets over the entire range of primary stresses.
The 25% and 50% mixture of re-cycle/total show
that lateral stress ratio is less than that for 100% pellets
over the entire range of primary stress, whereas 100% recycle
has a higher lateral stress than 100% pellets.
Therefore, the solids conveying rate with the addition of
re-cycle will be affected.
Bulk densities of the mixtures are also lower at
low stress, but are greater at higher stress. Therefore, the
combined effect of bulk density and lateral stress on solids
conveying is subject to the range of stress of the operation.
Lateral Stress Regression Functions
The following are the regression curves for the
data of lateral stress versus primary stress shown in Figure
5, and they are shown in Figure 6. A (0,0) intercept is
assumed (zero primary stress = zero lateral stress). The
trend line consistently used is a 6
th order polynomial. The
regression functions between 0 and 40 MPa do accurately
portray the data as can be seen comparing the data of
Figures 5 with the regression representations of Figure 6.
Therefore, the following equations are provided for
convenient accurate use of lateral stress data.
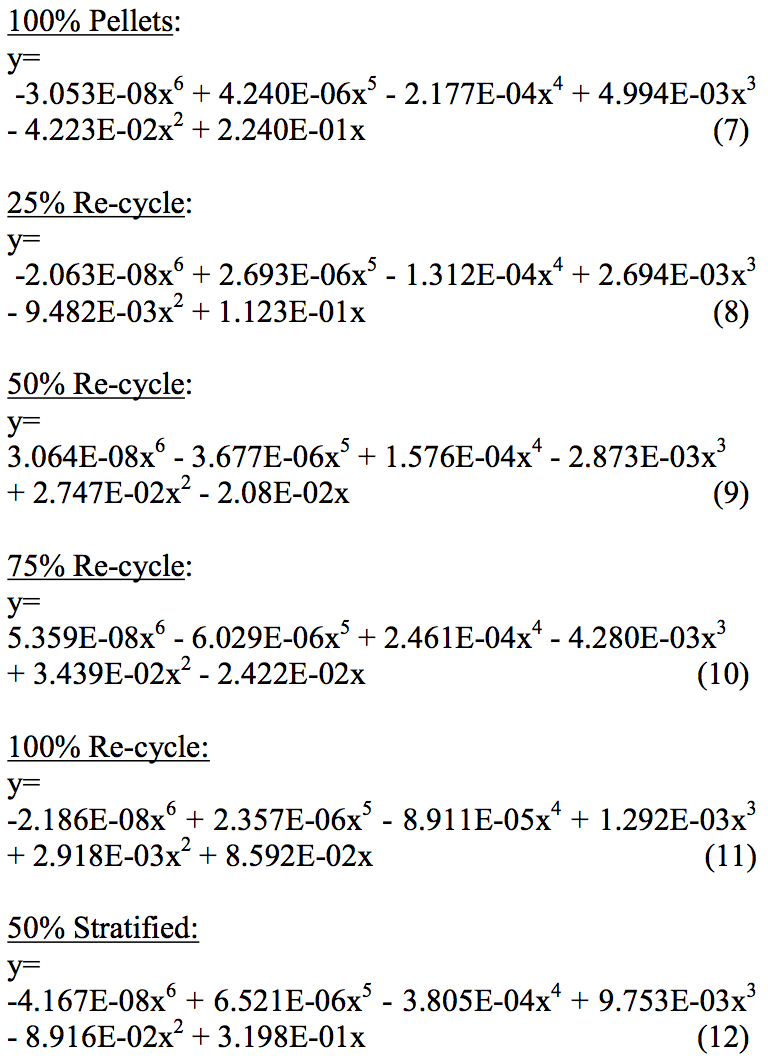
As with the bulk density regression functions,
above primary stress values of 40 MPa, error is likely.
Again, a linear extrapolation for primary stresses
moderately above 40 MPa could be used to extend the
useful range if need be.
Lateral Stress Ratio Functions
Lateral stress ratio [3,4] (the ratio of lateral stress
to primary stress) as a function of primary stress [2] is
easily obtained from the lateral stress polynomial
functions, Equations 7-12. The assumption that the data
have an intercept of 0,0 (zero lateral stress at zero primary stress) eliminates a constant term in Equations 7-12.
Therefore, Equations 7-12 can be divided by the primary
stress (x) and yield a finite result at x=0. This provides a
mathematical limit for the lateral stress ratio as primary
stress approaches zero. Previous work [2] for other
polymers has shown that those with lateral stress ratios of
0.3 or greater at low primary stress correlate with good
extrusion performance, and polymers with low lateral
stress ratios at low primary stress perform poorly.
Therefore, the limit of lateral stress ratio at zero stress is a
useful indicator of solids conveying performance.
Figure 7 shows the lateral stress ratio functions so
determined from Equations 7-12 with the mathematical
limit at zero primary stress (y intercept). The limits of
lateral stress ratio values at zero primary stress are unique
for the different ratios of pellets and re-cycle. It is highest
for the 100% Pellets (~0.22) and stratified 50% re-cycle
(~0.32). The mixtures of 25% re-cycle and 100% re-cycle
have a limit of lateral stress ratio of about 0.1. Pellets with
50% and 75% re-cycle show a limit near to zero.
The results of Figure 7 clearly indicate that
mixing virgin pellets and re-cycle produces non-linear
results. Also, the result is dependent on the level of
compressive stress. For example, resin with 25 % re-cycle
has lateral stress ratio less than that for 100% pellets at
primary stresses up to about 5 MPa. At 5 to 10 MPa stress
the lateral stress ratio for the mixture is about the same as
that for the 100% pellet resin. Above primary stress of 10
MPa, the lateral stress ratio of the 25% mixture is greater
than the pure pellets. The non-linearity and the reversing
of the effect can make processing difficult to manage and
that day to day operation would not be consistent since
mixture ratios likely could vary depending on the supply of
re-cycle or lack of mixing.
Figure 7 shows that the lateral stress ratio for 50%
and 75% re-cycle to always be lower than all other
samples, including pure pellets. Also, at zero primary
stress each of these two samples had zero lateral stress
ratio, whereas all of the other samples had finite values of
lateral stress at low primary stress.
Figure 7 also shows that the lateral stress ratio for
50% stratified pellets and re-cycle to have the greatest
variability of all the samples. Stratification of the recycle/pellet
mixture is inevitable, random, and difficult to
detect in the extruder. Stratification will, thereby, make
the lateral stress ratio inconsistent, and solids conveying
less predictable. Therefore, stratification should be
considered when flow instability occurs in the extrusion
process of mixtures of PET pellets and re-cycle.
Summary
Extrusion of PET pellet mixed with re-cycle
flakes in a single screw extruder is typically more difficult
than extruding PET pellets. Lateral stress and density for
mixtures of PET pellets and re-cycle flakes have been
shown here to depend on mixture fraction of re-cycle and
primary stress, and they are major factors in solids
conveying in single screw extruders. The dependence has
been shown to be non-linear and can depend on the level
of primary stress. Stratification is also likely to occur in
mixtures of re-cycle flakes and pellets, and this has been
shown to be a factor in lateral stress. The data here
provide quantitative evidence as to the sources of the
difficulty of extruding mixtures of PET pellets and PET recycle.
Hopefully, this will improve understanding of this
complex phenomenon and assist in the design of better
single screws for processing PET pellets with PET recycle.
Conclusions
1. For mixtures of PET pellets and PET re-cycle
flakes, the effect on lateral stress, as indicated by
the lateral stress ratio, is non-linear and dependent
on the level of primary stress. This greatly
complicates the solids conveying in single screw
extruders and makes consistent processing difficult.
2. The lateral stress of mixtures of PET pellets and
PET re-cycle flakes was measured to be a function
of the mixture ratio and primary stress.
3. Lateral stress for pure re-cycle flakes was measured
to be greater than for pure pellets. However, lateral
stress for mixtures with 50% and 75% re-cycle was
less than that for 100% pellets.
4. Lateral stress for a 50% stratified combination of
pellets and re-cycle flakes resulted in the greatest
values of lateral stress and the greatest variability of
lateral stress ratio.
5. Loose bulk density was measured to be greatest for
the pellets at about 0.75 g/cc. The addition of recycle
diminished the loose bulk density in
proportion to the amount of re-cycle. Pure re-cycle
has a loose bulk density of about 0.5 g/cc.
6. The bulk density of mixtures of PET pellets and
PET re-cycle flakes was measured in compression
as a function of mixture ratio and primary
compressive stress. The bulk density varied
between about 0.5 g/cc and 1.2 g/cc.
Acknowledgements
Very special thanks to son, Stephen J. Derezinski,
III of Platform Technology Ventures, Inc., for designing,
obtaining, building, and programming the computerized AD
data acquisition system for this work.
References
1. S. J. Derezinski 2009. “Laboratory Measurements
of Resin Bulk Specific gravity of PET and
LDPE,” ANTEC 2009 - Proceedings of the 67th
Annual Conference & Exhibition, Chicago, IL,
June 22-24, 2009, Society of Plastic Engineers,
pp. 136-141.
2. S. J. Derezinski 2010. “Measurements of Biaxial
Stresses During the Compression of Bulk Resin
Feed,” ANTEC 2010 - Proceedings of the 68th
Annual Conference & Exhibition, Orlando, Fl,
May 16-20, 2010, Society of Plastic Engineers,
pp. 617-622.
3. Chung, Chan I., Extrusion of Polymers Theory
and Practice, Hanser Publications, Munich, 2000,
pp. 161-162, 199-200.
4. M. A. Spalding, K. S. Hyun, and K. R. Hughes,
1996. “Stress Distribution in Solid Polymer
Compacts,” ANTEC 1996 - Proceedings of the
54th Annual Conference & Exhibition,
Indianapolis, IN, May 5-10, 1996, Society of
Plastic Engineers, pp. 191-198.
5. K. S. Hyun. and M. A. Spalding, 1997, “A New
Model for Solids Conveying in Plasticating
Extruders”, ANTEC 1997 - Proceedings of the
55th Annual Conference & Exhibition, 43, 1997.
Key Words
Stress, bulk density, solids conveying, single screw,
extrusion, pellets, PET re-cycle, PET, PET scrap, re-cycle
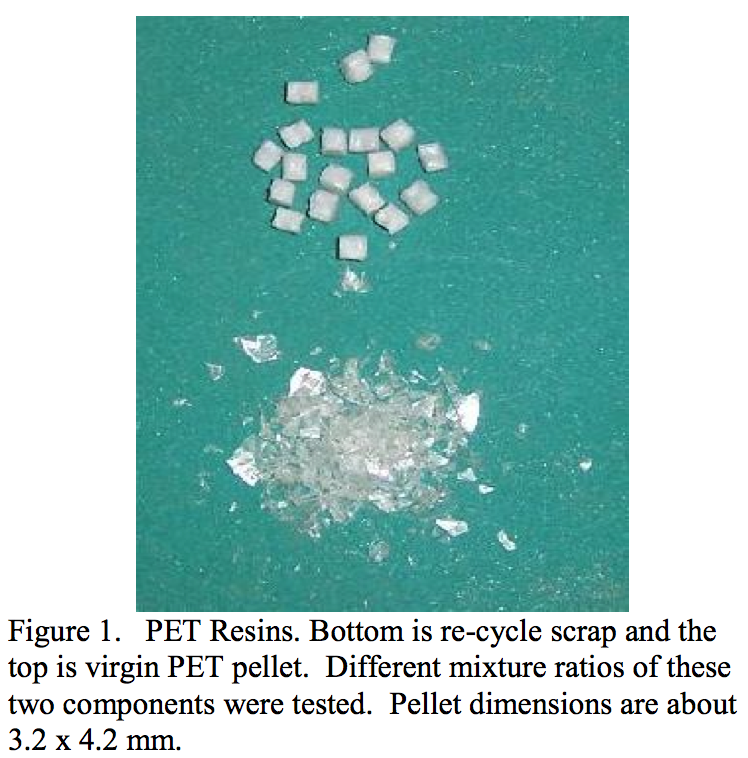
Figure 1. PET Resins. Bottom is re-cycle scrap and the top is virgin PET pellet. Different mixture ratios of these two components were tested. Pellet dimensions are about 3.2 x 4.2 mm.
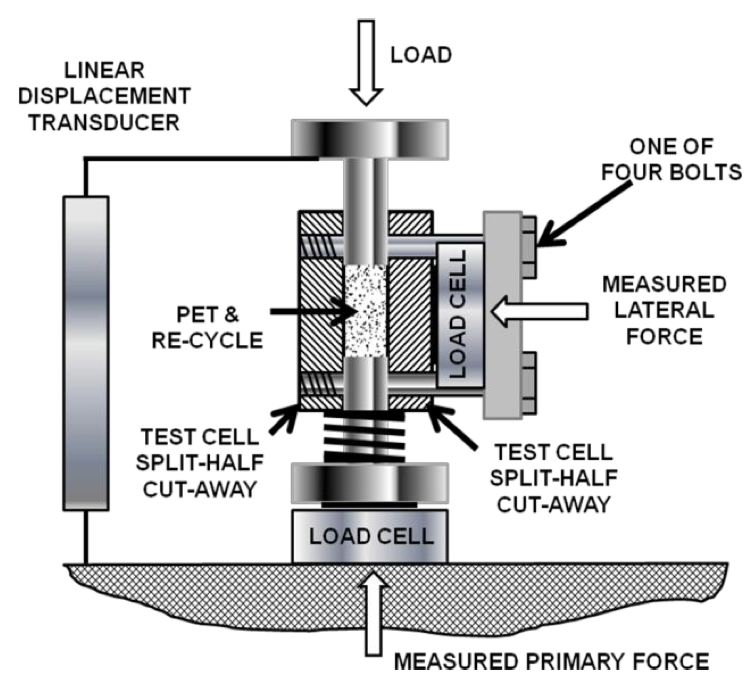
Figure 2. The Test Cell for Measuring the Primary and Lateral Forces During Compression of Bulk Resin Feed as a function of bulk density [1,2]. Diameter of the cylinder is 12.7 mm. The linear displacement transducer records the height of the sample to establish the evolving bulk density value and lateral area of force.
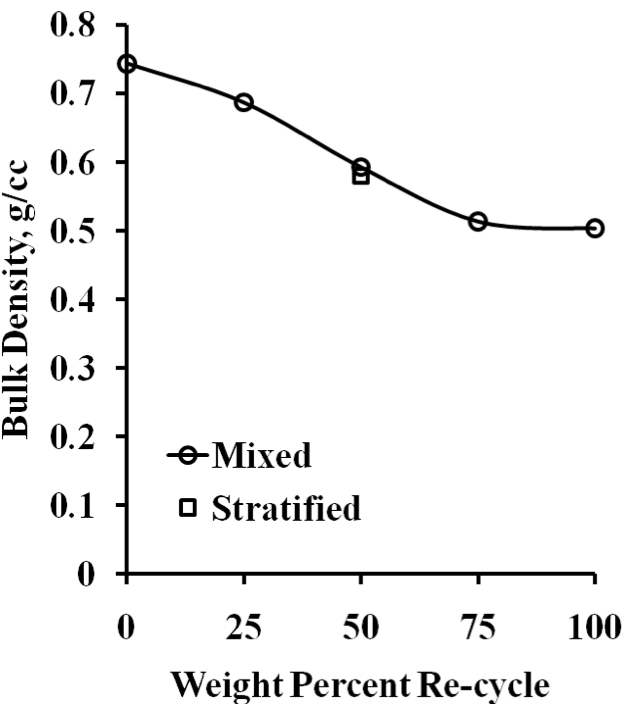
Figure 3. Bulk Density of PET Feed versus percent of Re-cycle Flakes. Values are those measured with the test cell before load is applied.
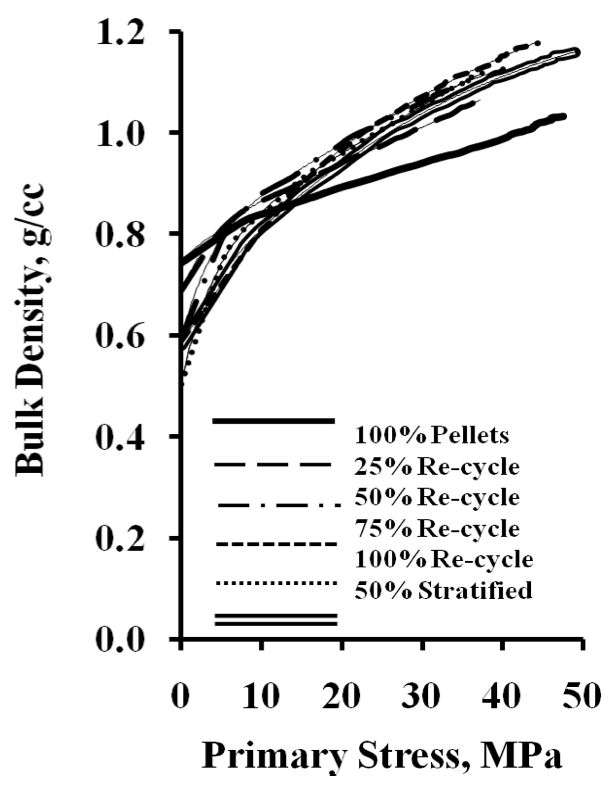
Figure 4. Data for Bulk Density of the Pellet/Re-cycle Mixtures vs. Primary Stress
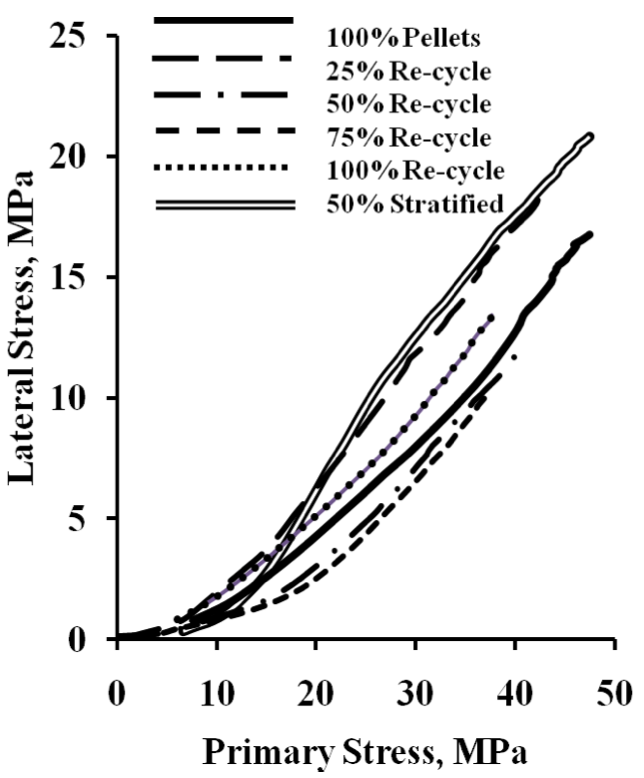
Figure 5. Raw Data for Lateral Stress for PET Pellets with Re-cycle vs. Primary Stress
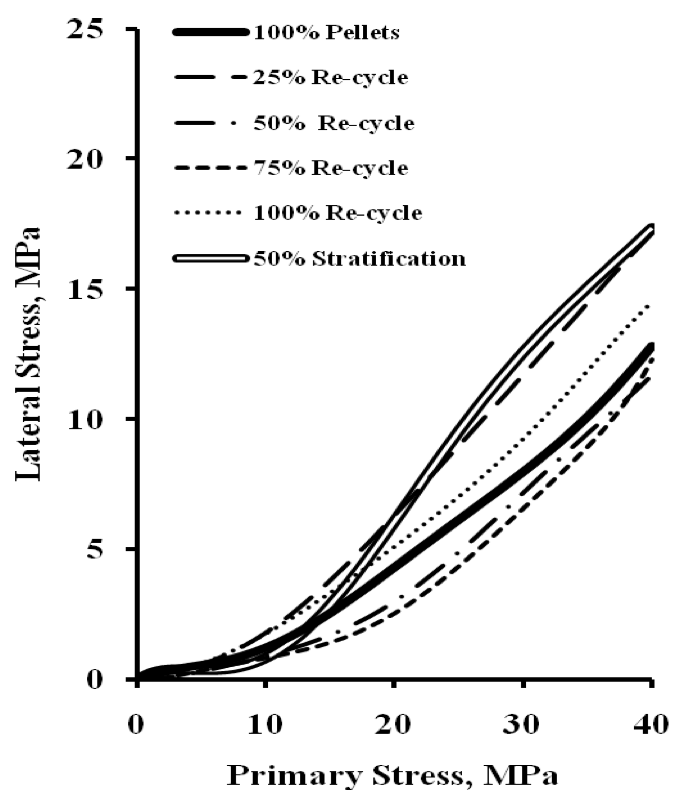
Figure 6. Regression Functions for Lateral Stress versus Primary Stress A 6th order polynomial is used for each set of data of Figure 5. Regression coefficients are greater than 0.999.
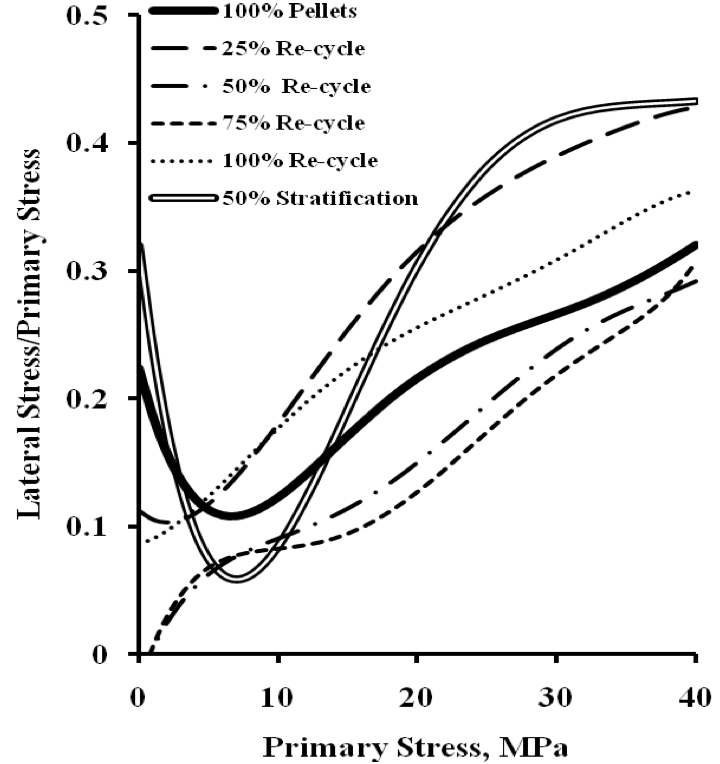
Figure 7. Lateral Stress Ratio Functions for PET/Re-cycle Mixtures
Return to
Paper of the Month.