Using Hypothesis Setting to Optimize the Troubleshooting Process for Single-Screw Plasticators
Mark A. Spalding, The Dow Chemical Company, Midland, MI
Gregory A. Campbell, Castle Research Associates, Jonesport, MEAbstract
The goal of all troubleshooting operations is to
restore the process to its original performance as quickly
as possible. This paper describes a process that is based
on developing hypotheses using verified data. Next, the
hypotheses are tested using properly developed
experiments. Once the root cause of the problem is
identified, the best technical solution is implemented.
Three case studies are presented.
Background
The goal of all troubleshooting operations is to
restore the process to its original performance as quickly
as possible. If the process is operational and producing a
high level of off specification product, then the
manufacturing costs can be very high. Restoring the line
to its original performance quickly will reduce costs by
eliminating some quality control operations and labor
wasted in making product that is not fit for use, reduction
in resin consumption, eliminating recycle due to off
specification product, and decreasing energy
consumption. Moreover if the line is inoperable due to the
defect, the downtime of the line can be extremely costly,
especially if the line is sold out. In this latter case, the
goal would be to bring the line back to production
operation as quickly as possible. Excellent overviews of
the troubleshooting process for extrusion systems were
provided by Gould [1] and Christie [2]. Procedures to
troubleshoot processes in general were outlined by Mager
[3] and Fogler and LeBlanc [4].
Troubleshooting a process can range from solving a
very simple problem such as replacing a malfunctioning
barrel heater to a very difficult flow problem that is very
complicated to diagnose. Collecting the proper
information on machine performance can minimize the
time required to restore the machine to its original
performance while reducing the cost of the
troubleshooting process. The machine owner will provide
details and information for the operation. Typically, the information will be a collection of facts, ideas on the root
cause, and data that are not relevant to the problem. The
troubleshooter must be able to listen to the information
provided and then sort the important facts from the nonrelevant
information. Often, several different solutions
will be possible. The best solution will be based on a
combination of the cost of lost production, the time and
cost to implement, machine owner acceptance, and the
risk associated with the modified process.
The first thing that a troubleshooter should do is talk
to the plant personnel and the process operators about the
defect. The operators in many cases witnessed the event
that caused the problem or they can provide the recent
history leading up to the failure. In some cases the
operator may have inadvertently caused the problem.
Interviewing the operator and having the operator assist
in the diagnosis of the problem can speed up the
troubleshooting process. After the interviews, the
troubleshooter must verify the accuracy of the
information. Verification of the information can be as
simple as viewing computer fault information on a control
panel to questioning events that are impossible to
reproduce or verify. The information that is verified will
become part of the basis for setting hypotheses on the
root cause of the problem.
The troubleshooter should obtain the performance
and modification history for the machine. This
information is typically available from electronic data
storage devices associated with the extruder, the machine
owner, maintenance personnel, and the operators. A
schematic for the screw is required for most
troubleshooting operations. Most screw vendors will
provide, as a courtesy, a diagram that shows the flow
channels of the screw. If these diagrams are not provided
during the original purchase, a diagram of the screw
should be made by plant personnel prior to installation.
Other required data include the rate, screw speed, motor
current, barrel temperature settings, and discharge
pressure and temperature. Additional sensor data are
occasionally available on some machines including
pressure measurements in the barrel, gear pump rate via
its rotation rate, and sensors specific to a process. The screw speed and motor current values displayed on the
control panel should be verified. For the screw speed,
counting the rotations of the screw at the back of the
gearbox for a time period must be performed to verify the
speed displayed on the panel. Most displays show the
screw speed within a 5% error, but for some processes the
screw speed display has been in error by a factor of 2.
Verifying the motor current is easily performed by an
electrician using a simple current meter. As a general
practice, all instruments and sensors should be verified
for accuracy.
All mechanical and electrical components should be
examined and verified that they are functioning properly.
These components include the solenoid valves for water
cooling systems, cooling water pumps, cooling fans,
electrical heaters, thermocouples and other temperature
sensors, pressure sensors, and gear pump operations.
Most extrusion processes measure the discharge
temperature using a thermocouple sensor positioned in
the transfer line downstream from the tip of the screw.
The thermocouple is often positioned into the polymer
stream about 1 cm past the inside wall of the transfer line.
This equipment configuration is the best for most
applications, but it can provide temperature measurements
that are considerably different from the actual resin
temperature [5]. The thermocouple measures the
temperature at the sensor junction, and this junction is
influenced by the temperature in its vicinity, including the
temperature of the resin, the temperature of the sensor
sheath, and the temperature of the transfer line. Since the
thermal conductivity for a metal is typically 300 times
higher than that for a polymer, thermal conduction is
more influenced by the surrounding metal than the
temperature of the resin in the transfer line. Thus, for a
transfer line that is controlled at a temperature less than
the bulk resin temperature, the thermocouple is going to
report a temperature that is less than the bulk temperature
of the resin. A better way to measure the discharge
temperature is by placing a hand-held thermocouple in the
resin discharging at the die opening. In order to mitigate
conduction of energy away from the junction, the
thermocouple sheath should be immersed in hot resin. If
the transfer line is relatively short with no significant
cooling or heating occurring in the line, then the
measured value should be close to the actual discharge
temperature from the extruder. For many systems where
the polymer flow is always inside lines, hand-held
measurements are not possible and the troubleshooter
must rely solely on the temperature measurement through
the transfer line. Hand-held temperature sensors that
measure the infrared (IR) radiation level from the resin
provide an excellent measure of relative temperatures, but
because of the difficulty in measuring the emissivity of
the polymer these devices are not as accurate as hand held thermocouple sensors.
Next, the troubleshooter should perform a basic
series of calculations for the screw and process. These
calculations include the rotational flow rate (or drag flow
rate) and pressure flow rate [6]. These calculations will
allow the troubleshooter to determine if the metering
section of the screw is the rate limiting section of the
process. The metering section of a flood-fed single-stage
screw or the first-stage metering section of a multi-stage
screw must control the rate for the process. If the
metering section is not rate controlling, then the extruder
will operate at reduced rates and has the potential to flow
surge and cause resin degradation products to occur in the
discharge [6,7]. For a multi-stage screw, if the first-stage
metering section does not control the rate then flow of
resin into a vent opening may occur. These calculations
are simple and will guide the troubleshooter to develop
appropriate hypotheses on the root cause of the process
defect. Other calculations that should be performed are
the compression rate and compression ratio. The
calculation of the compression ratio for a screw with a
constant lead length is as follows:

where C is the compression ratio, H is the channel depth
of the feed section, h is the depth of the metering channel.
The compression rate for the transition section of the
screw describes the rate that the channel depth changes as
the resin is transported through the section. The
compression rate is calculated as follows:


where R is the compression rate in the transition section,
M is the number of turns in the transition section, θ
b is the
helix angle at the barrel wall, L is the lead length, and Db
is the inside diameter of the barrel. The compression rate
and ratio should be within an acceptable range for the
resin processed.
To aid in the calculations and the relevance of their
application, the bulk density of the feedstock resin and
the shear viscosity should be measured. The shear
viscosity is needed for the pressure flow calculation while
the bulk density is needed to assess the compression ratio
and compression rate. For example, ground recycle
streams when added to pellets cause the bulk density of
the feedstock to decrease. If the bulk density of the feedstock is considerably less than just pellets, then the
compression ratio and compression rate should be
adjusted as follows:
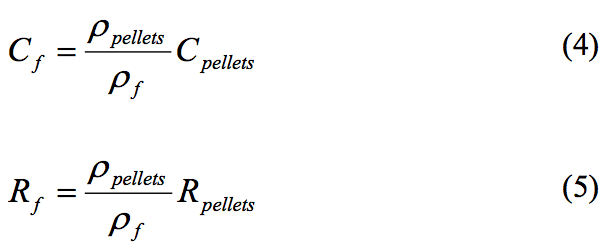
where Cpellets and Rpellets are the compression ratio and
compression rate used for a pellet feedstock, ρ
pellets is the
bulk density of the pellets at ambient conditions, ρ
f is the
bulk density of the feedstock mixture at ambient
conditions, and C
f and R
f are the compression ratio and
compression rate that should be used for the lower density
feedstock resin, respectively.
The specific energy inputted by the motor to the resin
should be calculated and compared to similar processes.
The power and specific energy inputted into the polymer
from the extruder screw are estimated using Equations (6)
and (7):
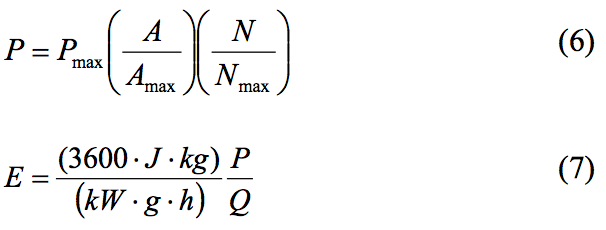
where P is the power that is dissipated in kW, P
max is the
nameplate power (kW) for the motor, A is the motor
current observed during the extrusion, A
max is the
nameplate motor current at full load, N is the screw speed
(rpm) during extrusion, and N
max is the maximum screw
speed (rpm) that the extruder is capable of running (with
full field voltage). After the power is computed, the
specific energy inputted to the resin from the screw, E, in
J/g is calculated using Equation (7) and the extrusion rate,
Q, in kg/h.
Often troubleshooting guides are provided by
equipment manufacturers for common problems. These
guides are helpful for many of the simpler problems
associated with the equipment. Some resin manufacturers
are an excellent resource for troubleshooting processing
problems that are specific to a resin. Subject matter
experts or extrusion consultants are also resources for
troubleshooting an extrusion process.
Spare parts for common components such as heaters
for barrels, transfer lines, and dies, thermocouples,
pressure transducers, drive belts, and fuses should be kept
in stock. Since the goal is to maintain the line operational at all times, keeping these low cost but necessary
components in stock can reduce the amount of downtime
due to simple failures. For operations where the resin is
abrasive or corrosive, a spare screw should be kept in
stock. As the screw wears in the extruder and the
performance decreases beyond an economic limit, then
the screw should be replaced with the spare screw and the
worn screw should be sent to a screw manufacturer for
refurbishment.
Hypothesis Setting and Problem Solving¶
With the plant interview information, verification of
the data, and the completion of the simple calculations, an
experienced troubleshooter will develop a set of
hypotheses for the root cause of the defect. After the
hypotheses are established a series of experiments need to
be developed that accept or reject the hypotheses. Once a
hypothesis is accepted via experimentation, then the next
step is to develop a technical solution to remove the
defect. Often more than one technical solution is possible.
The best technical solution will depend on the cost and
time to implement the solution, machine owner
acceptance, and the risk associated with the modified
process. An accepted hypothesis must drive the technical
solution. If a hypothesis is not accepted prior to
developing a technical solution, then the troubleshooter
may be working on the wrong problem and the defect
may not be eliminated from the process.
A hypothesis is a proposed explanation for an
observation. The hypothesis should be stated such that it
is clear and testable. For each hypothesis, an alternative
hypothesis should be stated that is accepted should the
original hypothesis be proven false. Developing
alternative hypotheses allows the troubleshooter to
quickly arrive at the defect while moving through a
complicated decision making process. The alternative
hypothesis provides a logical branch that directs the
troubleshooter to the root cause. Each troubleshooting
process should be developed with a set of alternative
hypotheses, design of experiments that exclude one or
more of the hypotheses, and then performing the
experiments such that definitive results occur [8]. At the
conclusion of this process, a new set of alternative
hypotheses may be required to continue the decision
making process.
As an example of an alternative hypothesis, a simple
case study is presented here. For this case, an extruder is
discharging degradation products into the product stream.
The hypothesis and alternative hypothesis statement is
“the metering section of the screw is not operating full
and not under pressure, creating regions where resin can
degrade, or the process is operating with the metering
section full and under pressure and the degradation products are coming in with the resin or generated in
some other section of the process.” This combination of
hypothesis is referred to here as the alternative hypothesis
since it allows for a decision to be made about where the
root cause occurs. Once an experiment is developed to
test the hypothesis, the troubleshooter can then focus the
next hypothesis and experiments looking at only one side
of the decision branch. Developing acceptable hypotheses
depends on validated information, a fundamental
knowledge of the process, and knowledge of the
properties of the resin.
Some of the most common root causes along with the
defects that they create are provided in Table 1. As shown
in this table, several defects can occur from the same root
cause, and a particular defect can be produced by several
root causes.
Once the root cause has been properly identified as
the source of the defect, a technical solution must be
devised that eliminates the root cause from the process.
As previously discussed, often more than one solution
exists. The best solution will depend on many factors
including the economic conditions for the plant and
product line, the cost the defect is creating at the plant,
the cost and time for implementing the technical
solutions, and the risk associated with each solution. Plant
personnel will ultimately decide on the best technical
solution for their plant.
Three case studies are presented next that
demonstrate the approach to troubleshooting problems.
The first two cases were developed with poor hypotheses
while the last case study had a problem that was solved
quickly using strong hypotheses and a strong
experimental plan for verification.
Case Study for the Design of a New Resin
A new general purpose polystyrene (GPPS) resin was
trialed at a customer’s injection molding plant as an
improvement over an incumbent resin manufactured by a
competitor. The new resin performed well in the process
except that it created parts with a 5% rejection rate due to
a splay defect. A photograph of a part with splay is shown
in Figure 1. The competitive resin was reported to run
well but with a lower defect rate. The plant manager
asked that the new resin be redesigned such that it had a
defect rate comparable to the competitive resin. Here the
hypothesis was that the new resin had a poor performance
relative to the incumbent resin, and the technical problem
to be solved was that the new resin needed to be modified
such that it performed as well as the incumbent resin. As
will be shown later, this technical problem was the wrong
problem to be solved.
Performance information for the incumbent resin was
missing from the early parts of the decision making
process. The decision that the technical problem was the
performance of the new resin was based on anecdotal
information from plant personnel on the performance of
the incumbent resin. That is, the plant personnel believed
that the reject level for parts made from the incumbent
resin was less than 5%. A statistical analysis of the part
defect rates was not performed. This lack of information
early in the process allowed the plant manager to pose a
poor technical solution without understanding the root
cause for the defect. A statistical analysis of the defect
rate indicated that the incumbent resin had a defect rate
that was statistically equivalent to the new resin.
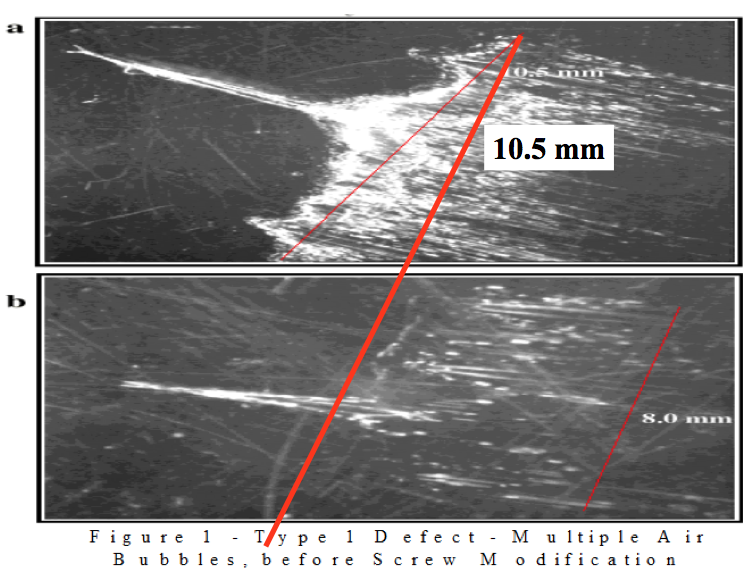
Figure 1. Microphotograph of the splay defect in a clear GPPS injection molded part. The flow direction was from the upper left to the lower right.
The primary and alternative hypotheses here are that
the injection molding machine process was creating the
defects in the parts, or the resin design was creating the
defects. The statistical analysis on the part defects molded
using the new and incumbent resins showed that the
resins were not the root cause for the defects. A small set
of exploratory experiments on the injection molding
machine allowed the development and acceptance of the
hypothesis that the screw design used in the plasticator
was not effective at melting the resin at high rates and
expelling entrained air out through the hopper. The new
technical problem to be solved was to increase the
melting capacity of the process and eliminate the
entrainment of air via process changes and screw
modifications. The technical details and the modifications
that were made to the screw were presented earlier [9].
When the modifications to the screw were finished, the
splay defects were eliminated and the capacity of the
plant was increased by about 14%.
This example clearly shows that developing and accepting a hypothesis based on accurate and complete
information is necessary for setting an acceptable
technical solution. If the plant manager could have
persuaded the resin manufacturer to develop a new resin
that was similar to the incumbent resin, then the defect
would still be there, the cost of the troubleshooting
process would have been extremely high, the supplier
would have incurred unnecessary development costs, and
a high level of defective parts would still have occurred
because the root cause would not have been removed.
Case Study for a Surface Blemish
A surface blemish on a specialty sheet product was
severely limiting the rate of the process. The blemish
appeared as a small (2 mm diameter) hemispherical pit or
crater in the surface. The level of surface defects could be
minimized but not totally eliminated by reducing the rate
of the process by 50%. A series of exploratory
experiments were performed and the defects could be
eliminated by decreasing the temperature of the last 6
barrel zones. The first 2 barrel zones were not adjusted so
as to not change the solids conveying behavior of the
resin. When large temperature changes (decreases in set
point temperatures of up to 50
oC) were made to these zones, the extrudate temperature was decreased by about 15
oC and the defects were totally eliminated. The hypothesis developed was that when the extrudate
temperature exceeded a specified value then surface
defects occurred. The technical solution was to develop a
process that discharged the resin at less than the specified
temperature and at high rate. The reason why the defects
occurred at higher discharge temperatures, however, was
unknown.
The resin was analyzed for moisture and other
volatiles that might cause a gas to be evolved at higher
temperatures. All analyses, however, did not indicate that
a gas was evolving or that the material was degrading.
These data are conflicting with the stated hypothesis. The
technical solution for this path was to design a screw with
a very deep metering section such that the extrudate is
discharged at as low a temperature as possible. Since the
material will discharge at increasing temperatures with
increasing screw speeds, the maximum rate will be
bounded when the extrudate exceeds the maximum
specified temperature.
In this case, the experiment developed to test the
hypothesis that high discharge temperatures create the
surface defects was flawed. The screw had a very low
compression ratio and compression rate. A better
hypothesis is that when the barrel zone temperatures are
decreased over the melting section, the temperature and
bulk density of the solid bed are decreased, allowing
entrained air to egress out through the hopper. To test this new hypothesis, the barrel zone temperatures over the
melting and metering sections were selectively changed
such that the melting zones were kept low while the
metering zones were increased. For these tests, the defects
in the sheet only appeared when the melting zone barrel
temperatures were high. The discharge temperature was
not a factor in controlling the defects. The new technical
solution was to design a screw with a high compression
rate and compression ratio such that entrained air can be
forced backward and out through the hopper.
A new screw was designed with a higher
compression rate and compression ratio. The new screw
was installed and the defects were totally eliminated. This
case study shows a poorly developed experiment that
incorrectly validated a poor hypothesis. If the second
experiment would have been performed first, the original
hypothesis of high discharge temperatures create surface
defects would have been invalidated. Clearly, the
experimental plan must be such that they definitively
validate or invalidate the hypothesis.
Case Study for a Profile Extrusion Process
A customer wanted to switch to a resin with a higher
modulus such that a large profile part could be made with
additional strength. The initial production trial was
performed and limited data were collected. The
information that came out of this trial was that the part
profile could not be maintained in specification, the
discharge temperature was higher than normal, and the
motor was operating at the maximum current load. Since
the trial did not last long, rate data were not collected.
The new resin was more viscous than the original resin.
Based on these very limited data, a second trial was
developed based on two hypotheses. The first hypothesis
was that the higher modulus of the new resin created a
blockage at the entry to a barrier melting section of the
screw. The blockage would cause the motor current to
increase dramatically and cause the specific rate for the
extruder to decrease. This type of screw defect was
presented previously [10]. The alternative hypothesis is
that a blockage did not occur and some other section of
the process other than the barrier section was the root
cause.
Preliminary work was performed prior to the trial and
included the measurement of the viscosities of both resins
around the discharge temperatures and the calculation of
the specific rotational flow rate for the metering channel
geometry. The specific rotational flow rate for the resins
were calculated at 11.3 kg/(h rpm). At the start of the
trial, the extruder and line were processing the original
resin at a specific rate of 11.0 kg/(h rpm), a value that is
consistent with the specific rotational rate. The part
profile was acceptable. Next the new resin with the higher modulus was added to the line. Within 30 minutes the
part profile dimensions were out of specification and the
motor was operating at near the maximum current limit. If
the first hypothesis is correct that a blockage is occurring
at the entry to the barrier melting section, then the
specific rate should be significantly less than the
calculated rotational rate of 11.3 kg/(h rpm). In this case,
the specific rate was measured at 11.1 kg/(h rpm), and
thus the first hypothesis that the barrier section was
causing a blockage was not valid. A second hypothesis
was developed that the higher viscosity of the new resin
caused too much energy to be dissipated, increasing the
motor load and the discharge temperature such that an
acceptable part profile could not be produced. To test this
hypothesis, the metering zone barrel temperatures were
slowly decreased in 10
oC increments [11] until the
cooling ability of the zones were at the maximum
capability. In order to maintain the motor torque at an
acceptable level, the temperature of the zones in the solids
conveying section were increased slightly. The extruder
was allowed to come to a steady-state operation. Within
45 minutes the part profile was on specification and the
extruder was operating at a specific rate of 11.0 kg/(h
rpm) and discharging at a temperature that was about
15
oC less than that at the start of the trial. The data
indicates that the second hypothesis is valid. The
technical solution for this case was to design a process
that discharges the new high-modulus resin at a lower
discharge temperature. In this case, plant personnel opted
for a new screw design with a deeper metering section to
decrease the energy dissipation level and decrease the
extrudate temperature.
This case study was developed with an alternative
hypothesis and then a second hypothesis, and the
experiments were designed properly to determine quickly
the root cause of the defect in the part profile. If the
hypotheses and experiments had not been developed
properly, the time required to troubleshoot the problem
would have increased or the project may have failed.
Conclusions
The time required to troubleshoot an extrusion
process or the plasticator on an injection molding
machine can be decreased by verifying operational data,
performing simple calculations, and developing strong
hypotheses. Next, the troubleshooter must develop
experiments that either validate or invalidate the
hypotheses. Once the root cause is determined, the best
technical solution will depend on many factors including
cost of lost production, the time and cost to implement,
machine owner acceptance, and the risk associated with
the modified process.
References
1. R.J. Gould, “Introduction – Basics of Extrusion
Troubleshooting,” in “The SPE Guide on Extrusion
Technology and Troubleshooting” Chapter I, Edited
by J. Vlachopoulos and J.R. Wagner, SPE, 2001.
2. A. Christie, “Troubleshooting the Extruder,” in “Film
Extrusion Manual,” Chapter 9, Edited by T.I. Butler
TAPPI Press, Atlanta, GA, 2005.
3. R.F. Mager, “Troubleshooting the Troubleshooting
Course or Debug D'Bugs,” Center for Effective
Performance, Atlanta, Georgia, 1983.
4. H.S. Fogler and S.E. LeBlanc, “Strategies for
Creative Problem Solving,” Prentice Hall PTR,
Upper Saddle River, New Jersey, 1995.
5. T.W. McCullough and M.A. Spalding, SPE-ANTEC
Tech. Papers, 42, 412 (1996).
6. M.A. Spalding, SPE-ANTEC Tech. Papers, 50, 329
(2004).
7. M.A. Spalding, J.R. Powers, P.A. Wagner, and K.S.
Hyun, SPE-ANTEC Tech. Papers, 46, 254 (2000).
8. J.R. Platt, Science, 146, 347 (1964).
9. M.A. Spalding and J.R. Powers, SPE-ANTEC Tech.
Papers, 55, 2463 (2009).
10. K.S. Hyun, M.A. Spalding, and J. Powers, SPEANTEC
Tech. Papers, 41, 293 (1995).
11. S.L. Crabtree, M.A. Spalding, and C.L. Pavlicek,
SPE-ANTEC Tech. Papers, 54, 1410 (2008).
12. Spalding, M.A., Dooley, J., and Hyun, K.S., SPEANTEC
Tech. Papers, 45, 190 (1999).
Key Words: Extrusion, Single-Screw, Troubleshooting,
Hypothesis Setting.
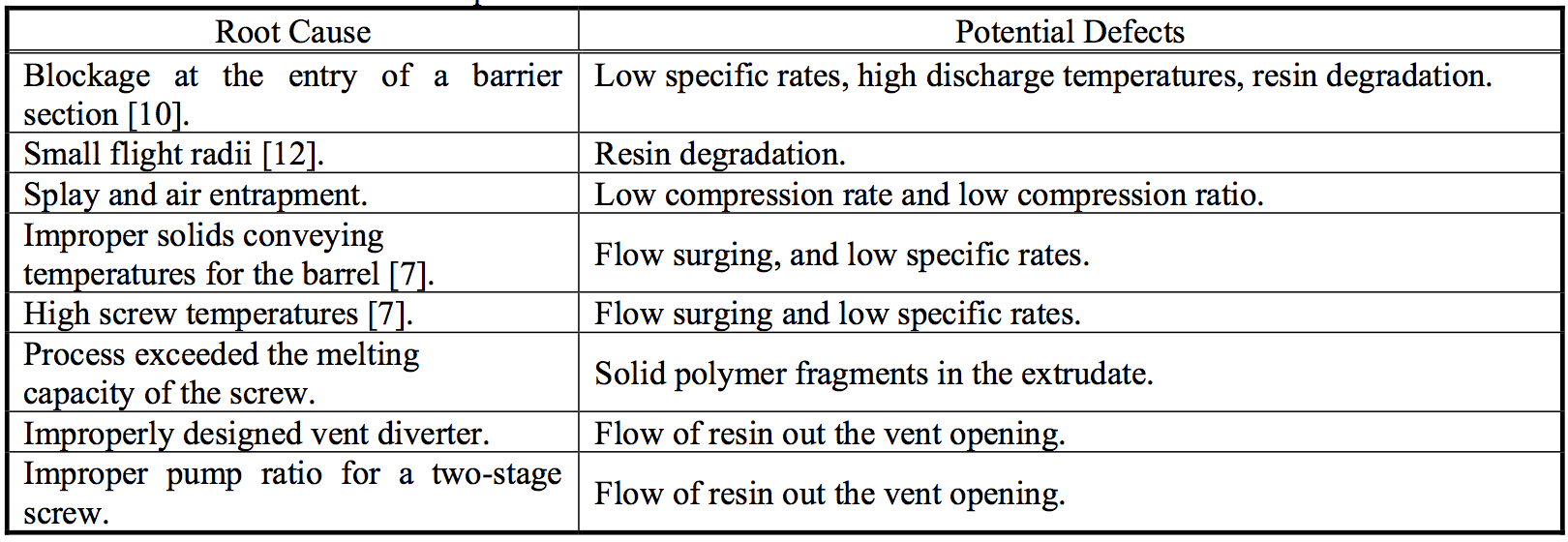
Table 1. Common root causes and potential defects.
Return to
Paper of the Month.